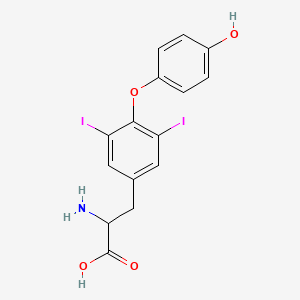
3,5-Diiodothyronine
Overview
Description
3,5-Diiodothyronine (T2) is a naturally occurring metabolite of thyroid hormones (THs), derived from the sequential deiodination of thyroxine (T4) and triiodothyronine (T3) . Its effects are dose-dependent, with high doses raising concerns about cardiac and hypothalamic-pituitary-thyroid (HPT) axis interactions, though these findings require further validation .
Preparation Methods
Metal Complex-Mediated Coupling
Copper-Complexed Diiodotyrosine Intermediate
The foundational method involves converting 3,5-diiodotyrosine (DIT) into a divalent metal complex, typically copper, to facilitate coupling with iodonium salts. As detailed in US3374269A , the process unfolds as follows:
-
Formation of Copper Complex :
-
Reaction with 4,4'-Dialkoxy-diphenyl-iodonium Salts :
-
Dealkylation :
Table 1: Optimization of Metal-Complex Method
Parameter | Condition | Yield (%) | Purity (%) |
---|---|---|---|
Metal Salt | CuSO₄ | 93–98 | 97+ |
Base | Triethylamine | 89 | 95 |
Reaction Temperature | 90°C | 95 | 98 |
Dealkylation Agent | HI/Acetic Acid | 95 | 99 |
Protective Group Strategies
Boc-Protected Tyrosine Pathway
PMC5607869 outlines a modern approach using tert-butoxycarbonyl (Boc) protection to enhance regioselectivity:
-
Boc Protection :
-
Iodination :
-
Coupling and Deprotection :
Key Insight : This method minimizes side reactions but requires costly reagents and multi-step purification.
Photolytic Decomposition of Thyroxine
Ultraviolet Light-Induced Deiodination
As reported in van der Walt and Cahnmann (PNAS) , thyroxine (T4) undergoes selective deiodination under UV light (300–340 nm):
-
Primary Photolysis :
-
Secondary Reactions :
Table 2: Photolysis Conditions and Outcomes
Wavelength (nm) | Time (min) | 3,5-T2 Yield (%) | Byproducts |
---|---|---|---|
>300 | 15 | 36 | T3 (27%), 3-T1 (10%) |
>340 | 40 | 13 | T3 (42%) |
Alternative Synthetic Routes
Direct Coupling of Diiodotyrosine Derivatives
EP302436B1 describes an industrial-scale process avoiding heavy metals:
-
One-Pot Coupling :
-
Purification :
Advantage : This method reduces genotoxic impurities and simplifies scalability.
Comparative Analysis of Methods
Table 3: Methodological Trade-offs
Method | Yield (%) | Purity (%) | Scalability | Cost |
---|---|---|---|---|
Metal Complex | 95 | 99 | High | Low |
Boc Protection | 76 | 98 | Moderate | High |
Photolysis | 36 | 90 | Low | Moderate |
Direct Coupling | 76 | 98.5 | High | Moderate |
Challenges and Innovations
-
Byproduct Formation : Photolysis and metal-mediated routes generate 3-T1 and residual copper, necessitating advanced chromatography .
-
Isotopic Labeling : PMC5607869 achieves 13C9-15N-labeled 3,5-T2 via Boc-protected tyrosine, enabling tracer studies.
-
Green Chemistry : Recent efforts replace HI with biodegradable dealkylation agents, though yields drop to 70–80% .
Chemical Reactions Analysis
Metal-Catalyzed Coupling Reaction
The primary industrial synthesis involves converting 3,5-diiodotyrosine to a divalent metal complex (e.g., copper) and reacting it with 4,4′-dialkoxy-diphenyl-iodonium salts under alkaline conditions . Key steps include:
-
Formation of metal-amino acid complexes :
-
Alkaline coupling with iodonium salts :
-
Dealkylation :
Alternative Biosynthetic Pathways
3,5-T2 is hypothesized to form via deiodination of T3 (triiodothyronine) by deiodinase enzymes (DIO1/DIO2), though direct in vitro evidence remains limited . Key findings:
-
In vivo studies : Rodent models show reduced serum 3,5-T2 levels after deiodinase inhibition (e.g., propylthiouracil) .
-
Human serum concentrations : 3,5-T2 averages 78 ± 9 pmol/L , correlating with its isomer 3,3′-T2 .
Table 2: Biosynthetic Evidence
Pathway | Enzyme Involved | Evidence Type | Reference |
---|---|---|---|
T3 → 3,5-T2 | DIO1/DIO2 | Indirect (PTU inhibition) | |
Thyroglobulin cleavage | Not confirmed | Theoretical |
Mitochondrial Interactions
3,5-T2 rapidly binds mitochondrial membranes, modulating energy metabolism:
-
Cytochrome c oxidase (COX) activation : At 1 pM, 3,5-T2 increases COX activity by 30–40% , counteracting ATP inhibition .
-
Uncoupling effects : Stimulates oxygen consumption in hepatocytes within 6 hours, independent of transcription .
Table 3: Mitochondrial Effects
Parameter | Effect Size | Experimental Model | Reference |
---|---|---|---|
COX activity | ↑ 30–40% at 1 pM | Rat liver | |
Oxygen consumption | ↑ 15% (resting metabolic rate) | Human trial |
Hydrolytic Stability
3,5-T2 resists spontaneous deiodination in neutral aqueous solutions but undergoes rapid degradation in strongly acidic/basic conditions .
Key Research Findings
-
Synthetic efficiency : The copper-catalyzed method achieves >90% yields without isolating intermediates .
-
Biosynthetic ambiguity : No in vitro enzymatic conversion of T3 to 3,5-T2 has been demonstrated, despite in vivo correlations .
-
Metabolic potency : 3,5-T2’s mitochondrial effects occur at picomolar concentrations, surpassing T3 in rapidity .
Scientific Research Applications
Metabolic Effects
1. Energy Metabolism and Weight Management
3,5-Diiodothyronine has been shown to increase resting metabolic rate and oxygen consumption in animal models. Research indicates that administration of 3,5-T2 can lead to significant reductions in body fat mass and serum cholesterol levels while promoting lipid metabolism. In a study involving diet-induced obese male C57BL/6J mice, 3,5-T2 administration resulted in a dose-dependent increase in metabolic rate and changes in gene expression related to lipid metabolism .
Table 1: Effects of this compound on Metabolic Parameters in Rodent Models
Parameter | Control Group | 3,5-T2 Treatment (0.25 μg) | 3,5-T2 Treatment (2.5 μg) |
---|---|---|---|
Body Weight (g) | 51.2 ± 1.2 | 50.6 ± 1.4 | 52.3 ± 1.6 |
Serum Cholesterol (mg/dL) | X | Reduced | Reduced |
Hepatic Triglycerides (mg/g) | X | Decreased | Decreased |
2. Hypolipidemic Effects
The compound has been proposed as a potential hypolipidemic agent due to its ability to enhance lipid metabolism without the typical side effects associated with other thyroid hormones like triiodothyronine (T3). Studies indicate that chronic administration of 3,5-T2 can significantly stimulate lipid β-oxidation and improve energy capacity in various tissues such as the heart and skeletal muscle .
Thyroid Function Modulation
1. Suppression of Thyroid Function
Research shows that this compound can suppress thyroid function by reducing the uptake of iodide and decreasing the activity of enzymes such as thyroperoxidase and deiodinases . This suppression is dose-dependent and may provide insights into managing conditions associated with excessive thyroid activity.
Table 2: Impact of this compound on Thyroid Function Parameters
Parameter | Control Group | 3,5-T2 Treatment (μg/100 g BW) |
---|---|---|
Serum TSH (μU/mL) | X | Reduced |
Serum T4 (ng/dL) | X | Reduced |
Thyroperoxidase Activity | X | Decreased |
Cardiovascular Implications
1. Cardiac Effects
While beneficial for metabolic regulation, this compound may also pose risks for cardiac health. Studies have indicated that treatment can lead to increased heart weights in rodent models, suggesting potential adverse effects on cardiac function . This highlights the need for careful monitoring when considering therapeutic applications.
Case Studies
Case Study: Chronic Administration in Rats
In a controlled experiment involving Wistar rats treated with varying doses of 3,5-T2 over a period of three months, researchers observed significant changes in metabolic rates and thyroid function markers. The results indicated an increase in oxygen consumption alongside a decrease in serum TSH levels, demonstrating the compound's dual role in enhancing metabolism while modulating thyroid activity .
Mechanism of Action
3,5-Diiodothyronine exerts its effects primarily through interactions with thyroid hormone receptors and mitochondrial pathways. It stimulates the TR-beta receptor for thyroid hormones, increasing energy expenditure. Additionally, it acts as an allosteric regulator of cytochrome c oxidase, enhancing mitochondrial respiration and energy production. These actions contribute to its metabolic effects and potential therapeutic benefits .
Comparison with Similar Compounds
Comparison with Other Diiodothyronines
Structural and Functional Differences
T2 belongs to a group of diiodothyronines, including 3,3′-T2 and 3′,5′-T2, which differ in iodine substitution positions (Table 1). Substitutions at the 3 and 5 positions on the inner phenolic ring are critical for T2’s metabolic activity, as demonstrated by its unique ability to stimulate 2,3-diphosphoglycerate (2,3-DPG) synthesis in erythrocytes, a feature absent in other analogs like 3,3′-T2 or thyronine .
Compound | Iodine Positions | Key Functions | Receptor Interaction |
---|---|---|---|
3,5-T2 (T2) | 3,5 (inner ring) | Mitochondrial activation, lipid oxidation, SIRT1-dependent metabolic regulation | Mitochondrial targets, SIRT1 |
3,3′-T2 | 3,3′ (outer ring) | Weak metabolic effects; unclear physiological role | Not well characterized |
3′,5′-T2 | 3′,5′ (outer ring) | Limited data; may influence age-related metabolic changes | Unknown |
Metabolic and Clinical Relevance
- T2 vs. 3,3′-T2 : While T2 reduces hepatic triglycerides and improves insulin sensitivity, 3,3′-T2 shows minimal impact on oxidative capacity in rat liver . Serum levels of 3,3′-T2 increase with age and illness but lack defined therapeutic roles .
- T2 vs. 3′,5′-T2 : 3′,5′-T2 concentrations decline with age in humans, but its metabolic contributions remain uncharacterized .
Comparison with Classical Thyroid Hormones (T3 and T4)
Mechanism of Action
- T2 : Acts via mitochondrial pathways (e.g., cytochrome c oxidase activation) and SIRT1-dependent deacetylation of PGC-1α/SREBP-1c, enhancing fatty acid oxidation and suppressing lipogenesis . Effects occur within 1 hour and are transcription-independent .
- T3/T4: Bind nuclear TRs to regulate gene expression, with effects manifesting after 24–72 hours .
Data Tables: Comparative Effects on Metabolic Parameters
Table 2. Metabolic Effects of T2 vs. T3 in Preclinical Models
Table 3. Serum Lipid Profile in Humans by T2 Levels
(Adapted from )
Parameter | Low T2 | Intermediate T2 | High T2 |
---|---|---|---|
LDL Cholesterol | ↑ | ↔ | ↓ |
HDL Cholesterol | ↓ | ↔ | ↑ |
Total Triglycerides | ↑ | ↔ | ↓ |
Biological Activity
3,5-Diiodothyronine (3,5-T2) is a biologically active metabolite of thyroid hormones that has garnered significant interest due to its metabolic effects and potential therapeutic applications. This article explores the biological activity of 3,5-T2, summarizing key research findings, case studies, and data tables that provide insights into its mechanisms of action.
Overview of this compound
This compound is an iodinated derivative of thyronine, which plays a crucial role in regulating metabolic processes. Unlike its more well-known counterparts, thyroxine (T4) and triiodothyronine (T3), 3,5-T2 exhibits distinct biological activities that can influence metabolism without the same level of thyroid-stimulating hormone (TSH) regulation.
1. Metabolic Effects:
3,5-T2 has been shown to increase resting metabolic rate (RMR) and oxygen consumption in various animal models. For instance, a study demonstrated that chronic administration of 3,5-T2 in Wistar rats led to increased oxygen consumption and a decrease in serum TSH and thyroid hormones (T4 and T3) levels in a dose-dependent manner .
2. Mitochondrial Activity:
Research indicates that 3,5-T2 enhances mitochondrial function by stimulating ATP synthesis and increasing mitochondrial respiration rates. It acts rapidly on mitochondrial ATP synthase activity within one hour of administration . The compound has been observed to increase mitochondrial calcium uptake, which further supports its role in enhancing mitochondrial activity .
Case Studies
- Obese Male Mice Study: In a study involving diet-induced obese male mice treated with 3,5-T2, researchers noted significant changes in hepatic gene expression related to lipid metabolism. The treatment resulted in reduced hepatic triglyceride levels and increased type I-deiodinase activity, indicating enhanced lipid metabolism . However, increased food intake compromised weight loss despite the desired metabolic effects.
- Hypothyroid Rats: Another study explored the effects of 3,5-T2 on hypothyroid rats subjected to a high-fat diet. The results showed that administration of 3,5-T2 led to anti-steatotic actions on the liver without significantly altering circulating levels of TSH or thyroid hormones .
Data Tables
Study | Model | Dosage | Key Findings |
---|---|---|---|
Lanni et al., 1996 | Wistar Rats | 25-75 μg/100 g BW | Increased RMR and oxygen consumption; reduced TSH and thyroid hormone levels |
Moreno et al., 2002 | Diet-Induced Obese Mice | 2.5 μg/g body weight | Enhanced lipid metabolism; reduced hepatic triglycerides; increased food intake |
Baur et al., 1997 | Hypothyroid Rats | Various doses | Increased D1 activity; decreased D2 activity; anti-steatotic effects |
Long-term Effects
Chronic administration of 3,5-T2 has been associated with improved energy capacity in various tissues including the heart and skeletal muscle. In cold-exposed rats treated with 3,5-T2, there was a significant increase in lipid β-oxidation and upregulation of mitochondrial enzymes involved in energy metabolism . This suggests that 3,5-T2 may play a role in enhancing thermogenic responses during cold exposure.
Q & A
Basic Research Questions
Q. What are the primary metabolic pathways influenced by T2, and how can researchers design experiments to isolate its effects from other thyroid hormones (e.g., T3, T4)?
- Methodological Answer : Use in vitro models (e.g., isolated mitochondria) to assess oxygen consumption rates via high-resolution respirometry, comparing T2-treated samples with controls. Employ pharmacological inhibitors (e.g., rotenone for Complex I) to pinpoint pathways. Pair this with LC-MS/MS to quantify T2 levels and rule out cross-reactivity with T3/T4 .
Q. What standardized assays are recommended for quantifying T2 in biological tissues, and what are their limitations?
- Methodological Answer : Liquid chromatography-tandem mass spectrometry (LC-MS/MS) is the gold standard due to its specificity and sensitivity. However, it requires expensive equipment and technical expertise. Validate assays using internal standards (e.g., isotopically labeled T2) to account for matrix effects . Immunoassays are less reliable due to potential cross-reactivity with structurally similar iodothyronines .
Q. How should researchers select appropriate animal models to study T2’s role in energy homeostasis?
- Methodological Answer : Use species with well-characterized thyroid signaling, such as rodents or killifish (Fundulus heteroclitus), which exhibit conserved responses to T2. Control for variables like age, diet, and circadian rhythms. Include sham-operated or thyroidectomized groups to isolate T2-specific effects .
Advanced Research Questions
Q. How can contradictory findings on T2’s thermogenic effects (e.g., mitochondrial uncoupling vs. enhanced efficiency) be resolved?
- Methodological Answer : Conduct comparative studies across tissues (liver vs. skeletal muscle) and species, using transcriptomic and metabolomic profiling to identify context-dependent mechanisms. Replicate conflicting experiments under identical conditions (e.g., dose, exposure duration) and apply meta-analysis to existing data .
Q. What experimental approaches can elucidate T2’s receptor-independent mechanisms, such as direct interactions with mitochondrial proteins?
- Methodological Answer : Employ proteomic techniques (e.g., affinity purification coupled with mass spectrometry) to identify T2-binding proteins. Validate findings using CRISPR/Cas9 knockout models for candidate proteins. Use fluorescently labeled T2 analogs for subcellular localization studies .
Q. How do researchers reconcile discrepancies in T2’s dose-response relationships observed in in vivo vs. in vitro studies?
- Methodological Answer : Perform pharmacokinetic studies to measure tissue-specific T2 bioavailability and clearance rates. Use physiologically based pharmacokinetic (PBPK) modeling to extrapolate in vitro effective concentrations to in vivo doses. Include time-course analyses to capture dynamic responses .
Q. Research Design & Data Synthesis
Q. What statistical methods are optimal for analyzing non-linear T2 effects in complex systems (e.g., hormesis)?
- Methodological Answer : Apply mixed-effects models to account for inter-individual variability. Use Bayesian approaches to handle sparse data and infer dose-response curves. Validate findings with bootstrapping or cross-validation to ensure robustness .
Q. How should researchers address the lack of standardized protocols for T2 administration in preclinical studies?
- Methodological Answer : Adopt the ARRIVE guidelines for experimental design transparency. Report detailed parameters (e.g., vehicle, administration route, dosing intervals) and validate T2 stability in solvents via HPLC. Collaborate via open-science platforms to establish consensus protocols .
Q. What strategies can mitigate confounding effects of endogenous T4/T3 degradation products in T2 studies?
- Methodological Answer : Use thyroid-blocking agents (e.g., propylthiouracil) to suppress endogenous hormone synthesis. Combine this with LC-MS/MS to monitor residual T4/T3 levels. Include control groups receiving deiodinase inhibitors to block T2 generation from T3 .
Q. Ethical & Translational Considerations
Q. How can researchers ensure ethical rigor when extrapolating T2’s metabolic benefits from animal models to human applications?
Properties
IUPAC Name |
2-amino-3-[4-(4-hydroxyphenoxy)-3,5-diiodophenyl]propanoic acid | |
---|---|---|
Source | PubChem | |
URL | https://pubchem.ncbi.nlm.nih.gov | |
Description | Data deposited in or computed by PubChem | |
InChI |
InChI=1S/C15H13I2NO4/c16-11-5-8(7-13(18)15(20)21)6-12(17)14(11)22-10-3-1-9(19)2-4-10/h1-6,13,19H,7,18H2,(H,20,21) | |
Source | PubChem | |
URL | https://pubchem.ncbi.nlm.nih.gov | |
Description | Data deposited in or computed by PubChem | |
InChI Key |
ZHSOTLOTTDYIIK-UHFFFAOYSA-N | |
Source | PubChem | |
URL | https://pubchem.ncbi.nlm.nih.gov | |
Description | Data deposited in or computed by PubChem | |
Canonical SMILES |
C1=CC(=CC=C1O)OC2=C(C=C(C=C2I)CC(C(=O)O)N)I | |
Source | PubChem | |
URL | https://pubchem.ncbi.nlm.nih.gov | |
Description | Data deposited in or computed by PubChem | |
Molecular Formula |
C15H13I2NO4 | |
Source | PubChem | |
URL | https://pubchem.ncbi.nlm.nih.gov | |
Description | Data deposited in or computed by PubChem | |
DSSTOX Substance ID |
DTXSID60862141 | |
Record name | 3,5-Diiodo-DL-thyronine | |
Source | EPA DSSTox | |
URL | https://comptox.epa.gov/dashboard/DTXSID60862141 | |
Description | DSSTox provides a high quality public chemistry resource for supporting improved predictive toxicology. | |
Molecular Weight |
525.08 g/mol | |
Source | PubChem | |
URL | https://pubchem.ncbi.nlm.nih.gov | |
Description | Data deposited in or computed by PubChem | |
Physical Description |
Solid | |
Record name | 3,5-Diiodothyronine | |
Source | Human Metabolome Database (HMDB) | |
URL | http://www.hmdb.ca/metabolites/HMDB0000582 | |
Description | The Human Metabolome Database (HMDB) is a freely available electronic database containing detailed information about small molecule metabolites found in the human body. | |
Explanation | HMDB is offered to the public as a freely available resource. Use and re-distribution of the data, in whole or in part, for commercial purposes requires explicit permission of the authors and explicit acknowledgment of the source material (HMDB) and the original publication (see the HMDB citing page). We ask that users who download significant portions of the database cite the HMDB paper in any resulting publications. | |
CAS No. |
534-51-0, 1041-01-6, 5563-89-3 | |
Record name | 3,5-Diiodo-DL-thyronine | |
Source | CAS Common Chemistry | |
URL | https://commonchemistry.cas.org/detail?cas_rn=534-51-0 | |
Description | CAS Common Chemistry is an open community resource for accessing chemical information. Nearly 500,000 chemical substances from CAS REGISTRY cover areas of community interest, including common and frequently regulated chemicals, and those relevant to high school and undergraduate chemistry classes. This chemical information, curated by our expert scientists, is provided in alignment with our mission as a division of the American Chemical Society. | |
Explanation | The data from CAS Common Chemistry is provided under a CC-BY-NC 4.0 license, unless otherwise stated. | |
Record name | 3,5-Diiodothyronine | |
Source | ChemIDplus | |
URL | https://pubchem.ncbi.nlm.nih.gov/substance/?source=chemidplus&sourceid=0000534510 | |
Description | ChemIDplus is a free, web search system that provides access to the structure and nomenclature authority files used for the identification of chemical substances cited in National Library of Medicine (NLM) databases, including the TOXNET system. | |
Record name | 3,5-Diiodothyronine | |
Source | DTP/NCI | |
URL | https://dtp.cancer.gov/dtpstandard/servlet/dwindex?searchtype=NSC&outputformat=html&searchlist=90469 | |
Description | The NCI Development Therapeutics Program (DTP) provides services and resources to the academic and private-sector research communities worldwide to facilitate the discovery and development of new cancer therapeutic agents. | |
Explanation | Unless otherwise indicated, all text within NCI products is free of copyright and may be reused without our permission. Credit the National Cancer Institute as the source. | |
Record name | NSC90468 | |
Source | DTP/NCI | |
URL | https://dtp.cancer.gov/dtpstandard/servlet/dwindex?searchtype=NSC&outputformat=html&searchlist=90468 | |
Description | The NCI Development Therapeutics Program (DTP) provides services and resources to the academic and private-sector research communities worldwide to facilitate the discovery and development of new cancer therapeutic agents. | |
Explanation | Unless otherwise indicated, all text within NCI products is free of copyright and may be reused without our permission. Credit the National Cancer Institute as the source. | |
Record name | 3,5-Diiodo-DL-thyronine | |
Source | EPA DSSTox | |
URL | https://comptox.epa.gov/dashboard/DTXSID60862141 | |
Description | DSSTox provides a high quality public chemistry resource for supporting improved predictive toxicology. | |
Record name | 3,5-diiodothyronine | |
Source | European Chemicals Agency (ECHA) | |
URL | https://echa.europa.eu/substance-information/-/substanceinfo/100.012.607 | |
Description | The European Chemicals Agency (ECHA) is an agency of the European Union which is the driving force among regulatory authorities in implementing the EU's groundbreaking chemicals legislation for the benefit of human health and the environment as well as for innovation and competitiveness. | |
Explanation | Use of the information, documents and data from the ECHA website is subject to the terms and conditions of this Legal Notice, and subject to other binding limitations provided for under applicable law, the information, documents and data made available on the ECHA website may be reproduced, distributed and/or used, totally or in part, for non-commercial purposes provided that ECHA is acknowledged as the source: "Source: European Chemicals Agency, http://echa.europa.eu/". Such acknowledgement must be included in each copy of the material. ECHA permits and encourages organisations and individuals to create links to the ECHA website under the following cumulative conditions: Links can only be made to webpages that provide a link to the Legal Notice page. | |
Record name | 3,5-DIIODOTHYRONINE, DL- | |
Source | FDA Global Substance Registration System (GSRS) | |
URL | https://gsrs.ncats.nih.gov/ginas/app/beta/substances/U1Y9GN485M | |
Description | The FDA Global Substance Registration System (GSRS) enables the efficient and accurate exchange of information on what substances are in regulated products. Instead of relying on names, which vary across regulatory domains, countries, and regions, the GSRS knowledge base makes it possible for substances to be defined by standardized, scientific descriptions. | |
Explanation | Unless otherwise noted, the contents of the FDA website (www.fda.gov), both text and graphics, are not copyrighted. They are in the public domain and may be republished, reprinted and otherwise used freely by anyone without the need to obtain permission from FDA. Credit to the U.S. Food and Drug Administration as the source is appreciated but not required. | |
Record name | 3,5-Diiodothyronine | |
Source | Human Metabolome Database (HMDB) | |
URL | http://www.hmdb.ca/metabolites/HMDB0000582 | |
Description | The Human Metabolome Database (HMDB) is a freely available electronic database containing detailed information about small molecule metabolites found in the human body. | |
Explanation | HMDB is offered to the public as a freely available resource. Use and re-distribution of the data, in whole or in part, for commercial purposes requires explicit permission of the authors and explicit acknowledgment of the source material (HMDB) and the original publication (see the HMDB citing page). We ask that users who download significant portions of the database cite the HMDB paper in any resulting publications. | |
Retrosynthesis Analysis
AI-Powered Synthesis Planning: Our tool employs the Template_relevance Pistachio, Template_relevance Bkms_metabolic, Template_relevance Pistachio_ringbreaker, Template_relevance Reaxys, Template_relevance Reaxys_biocatalysis model, leveraging a vast database of chemical reactions to predict feasible synthetic routes.
One-Step Synthesis Focus: Specifically designed for one-step synthesis, it provides concise and direct routes for your target compounds, streamlining the synthesis process.
Accurate Predictions: Utilizing the extensive PISTACHIO, BKMS_METABOLIC, PISTACHIO_RINGBREAKER, REAXYS, REAXYS_BIOCATALYSIS database, our tool offers high-accuracy predictions, reflecting the latest in chemical research and data.
Strategy Settings
Precursor scoring | Relevance Heuristic |
---|---|
Min. plausibility | 0.01 |
Model | Template_relevance |
Template Set | Pistachio/Bkms_metabolic/Pistachio_ringbreaker/Reaxys/Reaxys_biocatalysis |
Top-N result to add to graph | 6 |
Feasible Synthetic Routes
Disclaimer and Information on In-Vitro Research Products
Please be aware that all articles and product information presented on BenchChem are intended solely for informational purposes. The products available for purchase on BenchChem are specifically designed for in-vitro studies, which are conducted outside of living organisms. In-vitro studies, derived from the Latin term "in glass," involve experiments performed in controlled laboratory settings using cells or tissues. It is important to note that these products are not categorized as medicines or drugs, and they have not received approval from the FDA for the prevention, treatment, or cure of any medical condition, ailment, or disease. We must emphasize that any form of bodily introduction of these products into humans or animals is strictly prohibited by law. It is essential to adhere to these guidelines to ensure compliance with legal and ethical standards in research and experimentation.