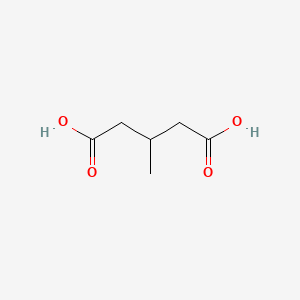
3-Methylglutaric acid
Overview
Description
Mechanism of Action
Target of Action
The primary target of 3-Methylglutaric acid is the 3-hydroxy-3-methylglutaryl-coenzyme A reductase (HMG-CoA reductase) . This enzyme plays a crucial role in the biosynthesis of cholesterol, acting as the rate-limiting step in the conversion of acetate to hydroxymethylglutaryl coenzyme A .
Mode of Action
This compound interferes with the enzymatic steps involved in the conversion of acetate to hydroxymethylglutaryl coenzyme A . It also inhibits the activity of HMG-CoA reductase, thereby affecting the biosynthesis of cholesterol .
Biochemical Pathways
This compound is an intermediate of the mitochondrial leucine catabolism . It accumulates when the conversion of 3-methylglutaconyl-coenzyme A (CoA) to 3-hydroxy-3-methylglutaryl-CoA (HMG-CoA) by the enzyme 3-methylglutaconyl-CoA hydratase is disturbed . This disturbance is the underlying cause in 3-Methylglutaconic aciduria type I .
Pharmacokinetics
It is known that in healthy individuals, this compound is found only in traces in the urine . In patients with 3-Methylglutaconic aciduria, concentrations can intermittently rise above 1,000 mmol/mol creatinine .
Result of Action
This compound can induce lipid oxidative damage and protein oxidative damage . It decreases the non-enzymatic antioxidant defenses in cerebral cortex supernatants, leading to oxidative stress in the cerebral cortex . It also diminishes synaptophysin levels and tau phosphorylation, and increases active caspase-3 content, indicative of cell damage .
Action Environment
The action of this compound can be influenced by environmental factors such as diet. For example, patients with AUH defect excrete even higher amounts of urinary this compound after a leucine-rich, or in general a protein-rich, meal . This suggests that dietary factors can influence the action, efficacy, and stability of this compound.
Biochemical Analysis
Biochemical Properties
3-Methylglutaric acid is involved in biochemical reactions primarily through its interaction with enzymes such as 3-hydroxy-3-methylglutaryl CoA lyase and 3-methylglutaconyl CoA hydratase . These enzymes are crucial in the leucine catabolic pathway. The interaction of this compound with these enzymes leads to its excretion in the urine of individuals with deficiencies in these enzymes. Additionally, this compound is synthesized via a novel mitochondrial biosynthetic pathway termed “the acetyl CoA diversion pathway,” which is initiated by defective electron transport chain function .
Cellular Effects
This compound has various effects on different types of cells and cellular processes. It influences cell function by impacting mitochondrial energy metabolism. In cells with compromised mitochondrial function, this compound accumulates, leading to disruptions in cellular metabolism and energy production . This accumulation can affect cell signaling pathways and gene expression, ultimately influencing cellular health and function.
Molecular Mechanism
The molecular mechanism of this compound involves its interaction with specific enzymes and biomolecules. It binds to and inhibits the activity of 3-hydroxy-3-methylglutaryl CoA lyase and 3-methylglutaconyl CoA hydratase, leading to the accumulation of this compound in the body . This inhibition disrupts the normal catabolic pathway of leucine, resulting in metabolic imbalances. Additionally, this compound can influence gene expression by altering the activity of transcription factors involved in mitochondrial function.
Temporal Effects in Laboratory Settings
In laboratory settings, the effects of this compound can change over time. The stability and degradation of this compound are important factors to consider. Over time, the accumulation of this compound can lead to long-term effects on cellular function, including chronic disruptions in energy metabolism and mitochondrial function . These effects can be observed in both in vitro and in vivo studies.
Dosage Effects in Animal Models
The effects of this compound vary with different dosages in animal models. At lower doses, this compound may have minimal impact on cellular function. At higher doses, it can lead to toxic effects, including severe disruptions in mitochondrial energy metabolism and cellular health . Threshold effects are observed, where a certain dosage level leads to significant adverse effects.
Metabolic Pathways
This compound is involved in several metabolic pathways, including the leucine catabolic pathway and the acetyl CoA diversion pathway . It interacts with enzymes such as 3-hydroxy-3-methylglutaryl CoA lyase and 3-methylglutaconyl CoA hydratase, affecting metabolic flux and metabolite levels. The accumulation of this compound can lead to metabolic imbalances and disruptions in energy production.
Transport and Distribution
Within cells and tissues, this compound is transported and distributed through specific transporters and binding proteins . These interactions influence its localization and accumulation within different cellular compartments. The transport and distribution of this compound are crucial for understanding its effects on cellular function and metabolism.
Subcellular Localization
This compound is localized within specific subcellular compartments, including the mitochondria . Its activity and function are influenced by its localization, with targeting signals and post-translational modifications directing it to specific organelles. The subcellular localization of this compound is essential for its role in mitochondrial energy metabolism and cellular health.
Preparation Methods
Synthetic Routes and Reaction Conditions
3-Methylglutaric acid can be synthesized through the oxidation of 3-methylglutaric aldehyde. The reaction typically involves the use of oxidizing agents such as potassium permanganate or chromium trioxide under acidic conditions . The reaction is carried out at room temperature, and the product is purified through recrystallization.
Industrial Production Methods
Industrial production of this compound involves the fermentation of specific strains of bacteria that can metabolize leucine. The fermentation process is optimized to maximize the yield of this compound, and the product is extracted and purified using standard biochemical techniques .
Chemical Reactions Analysis
Types of Reactions
3-Methylglutaric acid undergoes various chemical reactions, including:
Oxidation: It can be oxidized to form 3-methylglutaric aldehyde and further to this compound.
Reduction: It can be reduced to form 3-methylglutaric alcohol.
Substitution: It can undergo substitution reactions with halogens to form halogenated derivatives.
Common Reagents and Conditions
Oxidation: Potassium permanganate, chromium trioxide under acidic conditions.
Reduction: Sodium borohydride, lithium aluminum hydride.
Substitution: Halogens such as chlorine or bromine in the presence of a catalyst.
Major Products Formed
Oxidation: 3-Methylglutaric aldehyde, this compound.
Reduction: 3-Methylglutaric alcohol.
Substitution: Halogenated derivatives of this compound.
Scientific Research Applications
3-Methylglutaric acid has several scientific research applications:
Chemistry: It is used as a standard compound in nuclear magnetic resonance (NMR) spectroscopy for calibration purposes.
Biology: It is studied for its role in leucine catabolism and its involvement in metabolic disorders.
Medicine: Elevated levels of this compound are used as biomarkers for diagnosing metabolic diseases.
Comparison with Similar Compounds
Similar Compounds
3-Hydroxy-3-methylglutaric acid: Another intermediate in leucine catabolism.
3-Methylglutaconic acid: A closely related compound with similar metabolic roles.
Uniqueness
3-Methylglutaric acid is unique due to its specific role in leucine catabolism and its diagnostic significance in metabolic disorders. Unlike other similar compounds, it is a key biomarker for diagnosing 3-methylglutaconic aciduria and related metabolic diseases.
Properties
IUPAC Name |
3-methylpentanedioic acid | |
---|---|---|
Source | PubChem | |
URL | https://pubchem.ncbi.nlm.nih.gov | |
Description | Data deposited in or computed by PubChem | |
InChI |
InChI=1S/C6H10O4/c1-4(2-5(7)8)3-6(9)10/h4H,2-3H2,1H3,(H,7,8)(H,9,10) | |
Source | PubChem | |
URL | https://pubchem.ncbi.nlm.nih.gov | |
Description | Data deposited in or computed by PubChem | |
InChI Key |
XJMMNTGIMDZPMU-UHFFFAOYSA-N | |
Source | PubChem | |
URL | https://pubchem.ncbi.nlm.nih.gov | |
Description | Data deposited in or computed by PubChem | |
Canonical SMILES |
CC(CC(=O)O)CC(=O)O | |
Source | PubChem | |
URL | https://pubchem.ncbi.nlm.nih.gov | |
Description | Data deposited in or computed by PubChem | |
Molecular Formula |
C6H10O4 | |
Source | PubChem | |
URL | https://pubchem.ncbi.nlm.nih.gov | |
Description | Data deposited in or computed by PubChem | |
DSSTOX Substance ID |
DTXSID50211649 | |
Record name | 3-Methylglutaric acid | |
Source | EPA DSSTox | |
URL | https://comptox.epa.gov/dashboard/DTXSID50211649 | |
Description | DSSTox provides a high quality public chemistry resource for supporting improved predictive toxicology. | |
Molecular Weight |
146.14 g/mol | |
Source | PubChem | |
URL | https://pubchem.ncbi.nlm.nih.gov | |
Description | Data deposited in or computed by PubChem | |
Physical Description |
Solid | |
Record name | Methylglutaric acid | |
Source | Human Metabolome Database (HMDB) | |
URL | http://www.hmdb.ca/metabolites/HMDB0000752 | |
Description | The Human Metabolome Database (HMDB) is a freely available electronic database containing detailed information about small molecule metabolites found in the human body. | |
Explanation | HMDB is offered to the public as a freely available resource. Use and re-distribution of the data, in whole or in part, for commercial purposes requires explicit permission of the authors and explicit acknowledgment of the source material (HMDB) and the original publication (see the HMDB citing page). We ask that users who download significant portions of the database cite the HMDB paper in any resulting publications. | |
CAS No. |
626-51-7 | |
Record name | 3-Methylglutaric acid | |
Source | CAS Common Chemistry | |
URL | https://commonchemistry.cas.org/detail?cas_rn=626-51-7 | |
Description | CAS Common Chemistry is an open community resource for accessing chemical information. Nearly 500,000 chemical substances from CAS REGISTRY cover areas of community interest, including common and frequently regulated chemicals, and those relevant to high school and undergraduate chemistry classes. This chemical information, curated by our expert scientists, is provided in alignment with our mission as a division of the American Chemical Society. | |
Explanation | The data from CAS Common Chemistry is provided under a CC-BY-NC 4.0 license, unless otherwise stated. | |
Record name | 3-Methylglutaric acid | |
Source | ChemIDplus | |
URL | https://pubchem.ncbi.nlm.nih.gov/substance/?source=chemidplus&sourceid=0000626517 | |
Description | ChemIDplus is a free, web search system that provides access to the structure and nomenclature authority files used for the identification of chemical substances cited in National Library of Medicine (NLM) databases, including the TOXNET system. | |
Record name | 3-METHYLGLUTARIC ACID | |
Source | DTP/NCI | |
URL | https://dtp.cancer.gov/dtpstandard/servlet/dwindex?searchtype=NSC&outputformat=html&searchlist=14870 | |
Description | The NCI Development Therapeutics Program (DTP) provides services and resources to the academic and private-sector research communities worldwide to facilitate the discovery and development of new cancer therapeutic agents. | |
Explanation | Unless otherwise indicated, all text within NCI products is free of copyright and may be reused without our permission. Credit the National Cancer Institute as the source. | |
Record name | 3-Methylglutaric acid | |
Source | EPA DSSTox | |
URL | https://comptox.epa.gov/dashboard/DTXSID50211649 | |
Description | DSSTox provides a high quality public chemistry resource for supporting improved predictive toxicology. | |
Record name | 3-methylglutaric acid | |
Source | European Chemicals Agency (ECHA) | |
URL | https://echa.europa.eu/substance-information/-/substanceinfo/100.009.956 | |
Description | The European Chemicals Agency (ECHA) is an agency of the European Union which is the driving force among regulatory authorities in implementing the EU's groundbreaking chemicals legislation for the benefit of human health and the environment as well as for innovation and competitiveness. | |
Explanation | Use of the information, documents and data from the ECHA website is subject to the terms and conditions of this Legal Notice, and subject to other binding limitations provided for under applicable law, the information, documents and data made available on the ECHA website may be reproduced, distributed and/or used, totally or in part, for non-commercial purposes provided that ECHA is acknowledged as the source: "Source: European Chemicals Agency, http://echa.europa.eu/". Such acknowledgement must be included in each copy of the material. ECHA permits and encourages organisations and individuals to create links to the ECHA website under the following cumulative conditions: Links can only be made to webpages that provide a link to the Legal Notice page. | |
Record name | 3-METHYLGLUTARIC ACID | |
Source | FDA Global Substance Registration System (GSRS) | |
URL | https://gsrs.ncats.nih.gov/ginas/app/beta/substances/3Q0P190C7B | |
Description | The FDA Global Substance Registration System (GSRS) enables the efficient and accurate exchange of information on what substances are in regulated products. Instead of relying on names, which vary across regulatory domains, countries, and regions, the GSRS knowledge base makes it possible for substances to be defined by standardized, scientific descriptions. | |
Explanation | Unless otherwise noted, the contents of the FDA website (www.fda.gov), both text and graphics, are not copyrighted. They are in the public domain and may be republished, reprinted and otherwise used freely by anyone without the need to obtain permission from FDA. Credit to the U.S. Food and Drug Administration as the source is appreciated but not required. | |
Record name | Methylglutaric acid | |
Source | Human Metabolome Database (HMDB) | |
URL | http://www.hmdb.ca/metabolites/HMDB0000752 | |
Description | The Human Metabolome Database (HMDB) is a freely available electronic database containing detailed information about small molecule metabolites found in the human body. | |
Explanation | HMDB is offered to the public as a freely available resource. Use and re-distribution of the data, in whole or in part, for commercial purposes requires explicit permission of the authors and explicit acknowledgment of the source material (HMDB) and the original publication (see the HMDB citing page). We ask that users who download significant portions of the database cite the HMDB paper in any resulting publications. | |
Melting Point |
80 - 82 °C | |
Record name | Methylglutaric acid | |
Source | Human Metabolome Database (HMDB) | |
URL | http://www.hmdb.ca/metabolites/HMDB0000752 | |
Description | The Human Metabolome Database (HMDB) is a freely available electronic database containing detailed information about small molecule metabolites found in the human body. | |
Explanation | HMDB is offered to the public as a freely available resource. Use and re-distribution of the data, in whole or in part, for commercial purposes requires explicit permission of the authors and explicit acknowledgment of the source material (HMDB) and the original publication (see the HMDB citing page). We ask that users who download significant portions of the database cite the HMDB paper in any resulting publications. | |
Retrosynthesis Analysis
AI-Powered Synthesis Planning: Our tool employs the Template_relevance Pistachio, Template_relevance Bkms_metabolic, Template_relevance Pistachio_ringbreaker, Template_relevance Reaxys, Template_relevance Reaxys_biocatalysis model, leveraging a vast database of chemical reactions to predict feasible synthetic routes.
One-Step Synthesis Focus: Specifically designed for one-step synthesis, it provides concise and direct routes for your target compounds, streamlining the synthesis process.
Accurate Predictions: Utilizing the extensive PISTACHIO, BKMS_METABOLIC, PISTACHIO_RINGBREAKER, REAXYS, REAXYS_BIOCATALYSIS database, our tool offers high-accuracy predictions, reflecting the latest in chemical research and data.
Strategy Settings
Precursor scoring | Relevance Heuristic |
---|---|
Min. plausibility | 0.01 |
Model | Template_relevance |
Template Set | Pistachio/Bkms_metabolic/Pistachio_ringbreaker/Reaxys/Reaxys_biocatalysis |
Top-N result to add to graph | 6 |
Feasible Synthetic Routes
Disclaimer and Information on In-Vitro Research Products
Please be aware that all articles and product information presented on BenchChem are intended solely for informational purposes. The products available for purchase on BenchChem are specifically designed for in-vitro studies, which are conducted outside of living organisms. In-vitro studies, derived from the Latin term "in glass," involve experiments performed in controlled laboratory settings using cells or tissues. It is important to note that these products are not categorized as medicines or drugs, and they have not received approval from the FDA for the prevention, treatment, or cure of any medical condition, ailment, or disease. We must emphasize that any form of bodily introduction of these products into humans or animals is strictly prohibited by law. It is essential to adhere to these guidelines to ensure compliance with legal and ethical standards in research and experimentation.