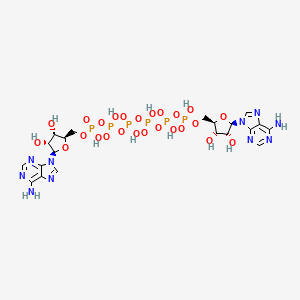
Diadenosine hexaphosphate
- Click on QUICK INQUIRY to receive a quote from our team of experts.
- With the quality product at a COMPETITIVE price, you can focus more on your research.
Overview
Description
Adenosine 5’-hexaphosphate 5’-ester with adenosine, commonly known as AP6A, is a dinucleoside polyphosphate. It consists of two adenosine molecules linked by a chain of six phosphate groups.
Preparation Methods
Synthetic Routes and Reaction Conditions
AP6A can be synthesized through enzymatic or chemical methods. One common enzymatic method involves the use of adenosine kinase and polyphosphate kinase to catalyze the formation of AP6A from adenosine and polyphosphate substrates. The reaction typically occurs under mild conditions, such as a pH of 7.5 and a temperature of 37°C .
Industrial Production Methods
In an industrial setting, AP6A can be produced using large-scale enzymatic reactors. These reactors maintain optimal conditions for the enzymatic reactions, including temperature, pH, and substrate concentrations. The product is then purified using techniques such as high-performance liquid chromatography (HPLC) to achieve high purity levels .
Chemical Reactions Analysis
Types of Reactions
AP6A undergoes various types of chemical reactions, including hydrolysis, phosphorylation, and complexation. Hydrolysis of AP6A can occur in the presence of specific hydrolases, leading to the formation of adenosine and inorganic phosphate .
Common Reagents and Conditions
Hydrolysis: Catalyzed by Nudix hydrolases, typically at physiological pH and temperature.
Phosphorylation: Can be achieved using kinases in the presence of ATP and magnesium ions.
Complexation: Forms complexes with metal ions such as magnesium and calcium under physiological conditions.
Major Products Formed
Hydrolysis: Adenosine and inorganic phosphate.
Phosphorylation: Higher-order polyphosphates and nucleoside triphosphates.
Complexation: Metal ion-AP6A complexes.
Scientific Research Applications
AP6A has a wide range of applications in scientific research:
Mechanism of Action
AP6A exerts its effects through interactions with specific molecular targets, such as Nudix hydrolases and P2X receptors. The compound binds to these targets, leading to the hydrolysis of AP6A and the subsequent release of adenosine and inorganic phosphate. This process can modulate various signaling pathways, including those involved in neurotransmitter release and vascular tone regulation .
Comparison with Similar Compounds
Similar Compounds
- Adenosine 5’-pentaphosphate (AP5A)
- Adenosine 5’-tetraphosphate (AP4A)
- Adenosine 5’-triphosphate (ATP)
Comparison
AP6A is unique due to its longer phosphate chain compared to similar compounds like AP5A and AP4A. This extended chain allows AP6A to form more stable complexes with metal ions and interact with a broader range of molecular targets. Additionally, AP6A has distinct physiological effects, such as its ability to modulate blood pressure and neurotransmitter release, which are not observed with shorter-chain polyphosphates .
Properties
CAS No. |
56983-23-4 |
---|---|
Molecular Formula |
C20H30N10O25P6 |
Molecular Weight |
996.3 g/mol |
IUPAC Name |
[[(2R,3S,4R,5R)-5-(6-aminopurin-9-yl)-3,4-dihydroxyoxolan-2-yl]methoxy-hydroxyphosphoryl] [[[[[(2R,3S,4R,5R)-5-(6-aminopurin-9-yl)-3,4-dihydroxyoxolan-2-yl]methoxy-hydroxyphosphoryl]oxy-hydroxyphosphoryl]oxy-hydroxyphosphoryl]oxy-hydroxyphosphoryl] hydrogen phosphate |
InChI |
InChI=1S/C20H30N10O25P6/c21-15-9-17(25-3-23-15)29(5-27-9)19-13(33)11(31)7(49-19)1-47-56(35,36)51-58(39,40)53-60(43,44)55-61(45,46)54-59(41,42)52-57(37,38)48-2-8-12(32)14(34)20(50-8)30-6-28-10-16(22)24-4-26-18(10)30/h3-8,11-14,19-20,31-34H,1-2H2,(H,35,36)(H,37,38)(H,39,40)(H,41,42)(H,43,44)(H,45,46)(H2,21,23,25)(H2,22,24,26)/t7-,8-,11-,12-,13-,14-,19-,20-/m1/s1 |
InChI Key |
PZCFFCOJNXGTIM-XPWFQUROSA-N |
Isomeric SMILES |
C1=NC(=C2C(=N1)N(C=N2)[C@H]3[C@@H]([C@@H]([C@H](O3)COP(=O)(O)OP(=O)(O)OP(=O)(O)OP(=O)(O)OP(=O)(O)OP(=O)(O)OC[C@@H]4[C@H]([C@H]([C@@H](O4)N5C=NC6=C(N=CN=C65)N)O)O)O)O)N |
SMILES |
C1=NC(=C2C(=N1)N(C=N2)C3C(C(C(O3)COP(=O)(O)OP(=O)(O)OP(=O)(O)OP(=O)(O)OP(=O)(O)OP(=O)(O)OCC4C(C(C(O4)N5C=NC6=C(N=CN=C65)N)O)O)O)O)N |
Canonical SMILES |
C1=NC(=C2C(=N1)N(C=N2)C3C(C(C(O3)COP(=O)(O)OP(=O)(O)OP(=O)(O)OP(=O)(O)OP(=O)(O)OP(=O)(O)OCC4C(C(C(O4)N5C=NC6=C(N=CN=C65)N)O)O)O)O)N |
56983-23-4 | |
physical_description |
Solid |
Synonyms |
Ap(6)A Ap6A diadenosine 5',5''''-P1,P6-hexaphosphate diadenosine hexaphosphate P1,P6-di(adenosine-5')hexaphosphate |
Origin of Product |
United States |
Retrosynthesis Analysis
AI-Powered Synthesis Planning: Our tool employs the Template_relevance Pistachio, Template_relevance Bkms_metabolic, Template_relevance Pistachio_ringbreaker, Template_relevance Reaxys, Template_relevance Reaxys_biocatalysis model, leveraging a vast database of chemical reactions to predict feasible synthetic routes.
One-Step Synthesis Focus: Specifically designed for one-step synthesis, it provides concise and direct routes for your target compounds, streamlining the synthesis process.
Accurate Predictions: Utilizing the extensive PISTACHIO, BKMS_METABOLIC, PISTACHIO_RINGBREAKER, REAXYS, REAXYS_BIOCATALYSIS database, our tool offers high-accuracy predictions, reflecting the latest in chemical research and data.
Strategy Settings
Precursor scoring | Relevance Heuristic |
---|---|
Min. plausibility | 0.01 |
Model | Template_relevance |
Template Set | Pistachio/Bkms_metabolic/Pistachio_ringbreaker/Reaxys/Reaxys_biocatalysis |
Top-N result to add to graph | 6 |
Feasible Synthetic Routes
Disclaimer and Information on In-Vitro Research Products
Please be aware that all articles and product information presented on BenchChem are intended solely for informational purposes. The products available for purchase on BenchChem are specifically designed for in-vitro studies, which are conducted outside of living organisms. In-vitro studies, derived from the Latin term "in glass," involve experiments performed in controlled laboratory settings using cells or tissues. It is important to note that these products are not categorized as medicines or drugs, and they have not received approval from the FDA for the prevention, treatment, or cure of any medical condition, ailment, or disease. We must emphasize that any form of bodily introduction of these products into humans or animals is strictly prohibited by law. It is essential to adhere to these guidelines to ensure compliance with legal and ethical standards in research and experimentation.