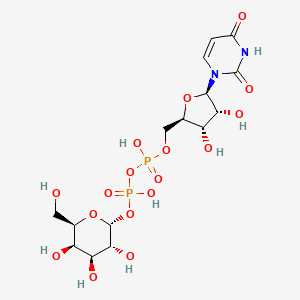
UDP-galactose
Overview
Description
Galactose-uridine-5’-diphosphate is a nucleoside diphosphate sugar that plays a crucial role in carbohydrate metabolism. It serves as a source of galactose in the synthesis of various biomolecules, including lipopolysaccharides, cerebrosides, and lactose . This compound is particularly important in the metabolism of nucleotide sugars and is involved in the production of polysaccharides .
Preparation Methods
Synthetic Routes and Reaction Conditions
Galactose-uridine-5’-diphosphate can be synthesized through enzymatic reactions involving uridine diphosphate-glucose dehydrogenase. This enzyme catalyzes the oxidation of uridine diphosphate-glucose to uridine diphosphate-glucuronic acid, which can then be converted to uridine diphosphate-galactose . The reaction conditions typically involve the presence of NAD+ as a cofactor and a suitable buffer system to maintain the pH .
Industrial Production Methods
In industrial settings, the production of galactose-uridine-5’-diphosphate often involves biocatalytic processes using microbial fermentation. The use of genetically engineered microorganisms that overexpress the necessary enzymes can enhance the yield and efficiency of production . The process may also include purification steps such as chromatographic techniques to obtain high-purity galactose-uridine-5’-diphosphate .
Chemical Reactions Analysis
Types of Reactions
Galactose-uridine-5’-diphosphate undergoes various chemical reactions, including epimerization, glycosylation, and hydrolysis .
Common Reagents and Conditions
Major Products
The major products formed from these reactions include uridine diphosphate-glucose, glycosylated proteins and lipids, and uridine monophosphate .
Scientific Research Applications
Galactose-uridine-5’-diphosphate has a wide range of scientific research applications:
Mechanism of Action
Galactose-uridine-5’-diphosphate exerts its effects through its role as a glycosyl donor in glycosylation reactions. It interacts with glycosyltransferases, which transfer the galactose moiety to specific acceptor molecules such as proteins and lipids . This process is crucial for the formation of glycoproteins and glycolipids, which play important roles in cell signaling, adhesion, and immune response .
Comparison with Similar Compounds
Similar Compounds
Uridine diphosphate-glucose: A precursor of glycogen and a substrate for the synthesis of polysaccharides containing glucose.
Uridine diphosphate-glucuronic acid: Involved in the detoxification of drugs and the synthesis of glycosaminoglycans.
Uridine diphosphate-N-acetylglucosamine: A substrate for the synthesis of glycoproteins and glycolipids.
Uniqueness
Galactose-uridine-5’-diphosphate is unique in its specific role as a galactose donor in glycosylation reactions. Unlike uridine diphosphate-glucose and uridine diphosphate-glucuronic acid, which are involved in broader metabolic pathways, galactose-uridine-5’-diphosphate is specifically required for the synthesis of galactose-containing biomolecules .
Properties
IUPAC Name |
[[(2R,3S,4R,5R)-5-(2,4-dioxopyrimidin-1-yl)-3,4-dihydroxyoxolan-2-yl]methoxy-hydroxyphosphoryl] [(2R,3R,4S,5R,6R)-3,4,5-trihydroxy-6-(hydroxymethyl)oxan-2-yl] hydrogen phosphate | |
---|---|---|
Source | PubChem | |
URL | https://pubchem.ncbi.nlm.nih.gov | |
Description | Data deposited in or computed by PubChem | |
InChI |
InChI=1S/C15H24N2O17P2/c18-3-5-8(20)10(22)12(24)14(32-5)33-36(28,29)34-35(26,27)30-4-6-9(21)11(23)13(31-6)17-2-1-7(19)16-15(17)25/h1-2,5-6,8-14,18,20-24H,3-4H2,(H,26,27)(H,28,29)(H,16,19,25)/t5-,6-,8+,9-,10+,11-,12-,13-,14-/m1/s1 | |
Source | PubChem | |
URL | https://pubchem.ncbi.nlm.nih.gov | |
Description | Data deposited in or computed by PubChem | |
InChI Key |
HSCJRCZFDFQWRP-ABVWGUQPSA-N | |
Source | PubChem | |
URL | https://pubchem.ncbi.nlm.nih.gov | |
Description | Data deposited in or computed by PubChem | |
Canonical SMILES |
C1=CN(C(=O)NC1=O)C2C(C(C(O2)COP(=O)(O)OP(=O)(O)OC3C(C(C(C(O3)CO)O)O)O)O)O | |
Source | PubChem | |
URL | https://pubchem.ncbi.nlm.nih.gov | |
Description | Data deposited in or computed by PubChem | |
Isomeric SMILES |
C1=CN(C(=O)NC1=O)[C@H]2[C@@H]([C@@H]([C@H](O2)COP(=O)(O)OP(=O)(O)O[C@@H]3[C@@H]([C@H]([C@H]([C@H](O3)CO)O)O)O)O)O | |
Source | PubChem | |
URL | https://pubchem.ncbi.nlm.nih.gov | |
Description | Data deposited in or computed by PubChem | |
Molecular Formula |
C15H24N2O17P2 | |
Source | PubChem | |
URL | https://pubchem.ncbi.nlm.nih.gov | |
Description | Data deposited in or computed by PubChem | |
DSSTOX Substance ID |
DTXSID60903962 | |
Record name | Uridine diphosphate galactose | |
Source | EPA DSSTox | |
URL | https://comptox.epa.gov/dashboard/DTXSID60903962 | |
Description | DSSTox provides a high quality public chemistry resource for supporting improved predictive toxicology. | |
Molecular Weight |
566.30 g/mol | |
Source | PubChem | |
URL | https://pubchem.ncbi.nlm.nih.gov | |
Description | Data deposited in or computed by PubChem | |
Physical Description |
Disodium salt: White powder; [Sigma-Aldrich MSDS], Solid | |
Record name | Uridine diphosphate galactose | |
Source | Haz-Map, Information on Hazardous Chemicals and Occupational Diseases | |
URL | https://haz-map.com/Agents/18589 | |
Description | Haz-Map® is an occupational health database designed for health and safety professionals and for consumers seeking information about the adverse effects of workplace exposures to chemical and biological agents. | |
Explanation | Copyright (c) 2022 Haz-Map(R). All rights reserved. Unless otherwise indicated, all materials from Haz-Map are copyrighted by Haz-Map(R). No part of these materials, either text or image may be used for any purpose other than for personal use. Therefore, reproduction, modification, storage in a retrieval system or retransmission, in any form or by any means, electronic, mechanical or otherwise, for reasons other than personal use, is strictly prohibited without prior written permission. | |
Record name | Uridine diphosphategalactose | |
Source | Human Metabolome Database (HMDB) | |
URL | http://www.hmdb.ca/metabolites/HMDB0000302 | |
Description | The Human Metabolome Database (HMDB) is a freely available electronic database containing detailed information about small molecule metabolites found in the human body. | |
Explanation | HMDB is offered to the public as a freely available resource. Use and re-distribution of the data, in whole or in part, for commercial purposes requires explicit permission of the authors and explicit acknowledgment of the source material (HMDB) and the original publication (see the HMDB citing page). We ask that users who download significant portions of the database cite the HMDB paper in any resulting publications. | |
CAS No. |
2956-16-3 | |
Record name | UDP-Galactose | |
Source | CAS Common Chemistry | |
URL | https://commonchemistry.cas.org/detail?cas_rn=2956-16-3 | |
Description | CAS Common Chemistry is an open community resource for accessing chemical information. Nearly 500,000 chemical substances from CAS REGISTRY cover areas of community interest, including common and frequently regulated chemicals, and those relevant to high school and undergraduate chemistry classes. This chemical information, curated by our expert scientists, is provided in alignment with our mission as a division of the American Chemical Society. | |
Explanation | The data from CAS Common Chemistry is provided under a CC-BY-NC 4.0 license, unless otherwise stated. | |
Record name | Uridine diphosphate galactose | |
Source | ChemIDplus | |
URL | https://pubchem.ncbi.nlm.nih.gov/substance/?source=chemidplus&sourceid=0002956163 | |
Description | ChemIDplus is a free, web search system that provides access to the structure and nomenclature authority files used for the identification of chemical substances cited in National Library of Medicine (NLM) databases, including the TOXNET system. | |
Record name | Galactose-uridine-5'-diphosphate | |
Source | DrugBank | |
URL | https://www.drugbank.ca/drugs/DB03501 | |
Description | The DrugBank database is a unique bioinformatics and cheminformatics resource that combines detailed drug (i.e. chemical, pharmacological and pharmaceutical) data with comprehensive drug target (i.e. sequence, structure, and pathway) information. | |
Explanation | Creative Common's Attribution-NonCommercial 4.0 International License (http://creativecommons.org/licenses/by-nc/4.0/legalcode) | |
Record name | Uridine diphosphate galactose | |
Source | EPA DSSTox | |
URL | https://comptox.epa.gov/dashboard/DTXSID60903962 | |
Description | DSSTox provides a high quality public chemistry resource for supporting improved predictive toxicology. | |
Record name | URIDINE 5'-DIPHOSPHOGALACTOSE | |
Source | FDA Global Substance Registration System (GSRS) | |
URL | https://gsrs.ncats.nih.gov/ginas/app/beta/substances/O2HY4WY2W1 | |
Description | The FDA Global Substance Registration System (GSRS) enables the efficient and accurate exchange of information on what substances are in regulated products. Instead of relying on names, which vary across regulatory domains, countries, and regions, the GSRS knowledge base makes it possible for substances to be defined by standardized, scientific descriptions. | |
Explanation | Unless otherwise noted, the contents of the FDA website (www.fda.gov), both text and graphics, are not copyrighted. They are in the public domain and may be republished, reprinted and otherwise used freely by anyone without the need to obtain permission from FDA. Credit to the U.S. Food and Drug Administration as the source is appreciated but not required. | |
Record name | Uridine diphosphategalactose | |
Source | Human Metabolome Database (HMDB) | |
URL | http://www.hmdb.ca/metabolites/HMDB0000302 | |
Description | The Human Metabolome Database (HMDB) is a freely available electronic database containing detailed information about small molecule metabolites found in the human body. | |
Explanation | HMDB is offered to the public as a freely available resource. Use and re-distribution of the data, in whole or in part, for commercial purposes requires explicit permission of the authors and explicit acknowledgment of the source material (HMDB) and the original publication (see the HMDB citing page). We ask that users who download significant portions of the database cite the HMDB paper in any resulting publications. | |
Retrosynthesis Analysis
AI-Powered Synthesis Planning: Our tool employs the Template_relevance Pistachio, Template_relevance Bkms_metabolic, Template_relevance Pistachio_ringbreaker, Template_relevance Reaxys, Template_relevance Reaxys_biocatalysis model, leveraging a vast database of chemical reactions to predict feasible synthetic routes.
One-Step Synthesis Focus: Specifically designed for one-step synthesis, it provides concise and direct routes for your target compounds, streamlining the synthesis process.
Accurate Predictions: Utilizing the extensive PISTACHIO, BKMS_METABOLIC, PISTACHIO_RINGBREAKER, REAXYS, REAXYS_BIOCATALYSIS database, our tool offers high-accuracy predictions, reflecting the latest in chemical research and data.
Strategy Settings
Precursor scoring | Relevance Heuristic |
---|---|
Min. plausibility | 0.01 |
Model | Template_relevance |
Template Set | Pistachio/Bkms_metabolic/Pistachio_ringbreaker/Reaxys/Reaxys_biocatalysis |
Top-N result to add to graph | 6 |
Feasible Synthetic Routes
Disclaimer and Information on In-Vitro Research Products
Please be aware that all articles and product information presented on BenchChem are intended solely for informational purposes. The products available for purchase on BenchChem are specifically designed for in-vitro studies, which are conducted outside of living organisms. In-vitro studies, derived from the Latin term "in glass," involve experiments performed in controlled laboratory settings using cells or tissues. It is important to note that these products are not categorized as medicines or drugs, and they have not received approval from the FDA for the prevention, treatment, or cure of any medical condition, ailment, or disease. We must emphasize that any form of bodily introduction of these products into humans or animals is strictly prohibited by law. It is essential to adhere to these guidelines to ensure compliance with legal and ethical standards in research and experimentation.