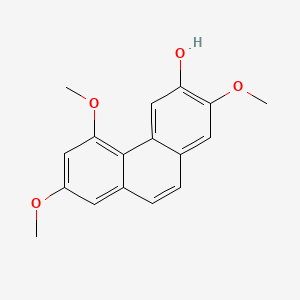
Batatasin I
Overview
Description
Mechanism of Action
Target of Action
Batatasin I, a naturally occurring phenanthrene derivative, primarily targets α-glucosidase and cyclooxygenase-2 (COX-2) . α-glucosidase is an enzyme that plays a crucial role in carbohydrate digestion, and its inhibition can help manage postprandial hyperglycemia, a common issue in diabetes . COX-2 is an enzyme that plays a key role in the generation of prostaglandins, which are involved in inflammation and pain .
Biochemical Analysis
Biochemical Properties
Batatasin I plays a crucial role in biochemical reactions, particularly as an inhibitor of enzymes involved in inflammation and glucose metabolism. It has been shown to inhibit cyclooxygenase-2 (COX-2) dependent prostaglandin D2 generation and 5-lipoxygenase (5-LOX) dependent leukotriene C4 generation in a dose-dependent manner . Additionally, this compound interacts with α-glucosidase, inhibiting its activity in a reversible and noncompetitive manner . These interactions highlight the compound’s potential as an anti-inflammatory and antidiabetic agent.
Cellular Effects
This compound exerts various effects on different cell types and cellular processes. In bone marrow-derived mast cells, this compound inhibits the generation of prostaglandin D2 and leukotriene C4, as well as the degranulation reaction . This compound also influences cell signaling pathways by reducing the expression of COX-2 protein levels . Furthermore, this compound’s inhibition of α-glucosidase suggests its potential to modulate glucose metabolism and cellular energy balance .
Molecular Mechanism
At the molecular level, this compound exerts its effects through specific binding interactions with enzymes and other biomolecules. It inhibits COX-2 and 5-LOX by binding to their active sites, thereby reducing the production of inflammatory mediators . Additionally, this compound’s interaction with α-glucosidase involves binding to the enzyme’s active site, leading to a decrease in its catalytic activity . These molecular interactions underscore the compound’s potential as a therapeutic agent.
Temporal Effects in Laboratory Settings
In laboratory settings, the effects of this compound have been observed to change over time. The compound’s stability and degradation have been studied, revealing that it maintains its inhibitory activity against COX-2 and 5-LOX over extended periods . Long-term studies have also shown that this compound can sustain its anti-inflammatory effects in vitro and in vivo . These findings suggest that this compound has a stable and lasting impact on cellular function.
Dosage Effects in Animal Models
The effects of this compound vary with different dosages in animal models. At lower doses, the compound effectively inhibits COX-2 and 5-LOX activity without causing significant adverse effects . At higher doses, this compound may exhibit toxic effects, including potential damage to liver and kidney tissues . These dosage-dependent effects highlight the importance of determining optimal therapeutic doses for safe and effective use.
Metabolic Pathways
This compound is involved in several metabolic pathways, particularly those related to inflammation and glucose metabolism. It interacts with enzymes such as COX-2, 5-LOX, and α-glucosidase, modulating their activity and influencing metabolic flux . These interactions can lead to changes in metabolite levels, such as reduced production of prostaglandins and leukotrienes . Understanding these metabolic pathways is crucial for elucidating the compound’s therapeutic potential.
Transport and Distribution
Within cells and tissues, this compound is transported and distributed through interactions with specific transporters and binding proteins. These interactions facilitate the compound’s localization to target sites, such as inflammatory cells and tissues . The distribution of this compound within the body can influence its therapeutic efficacy and potential side effects.
Subcellular Localization
This compound’s subcellular localization plays a significant role in its activity and function. The compound is directed to specific compartments or organelles through targeting signals and post-translational modifications . This localization allows this compound to exert its effects precisely where needed, such as within inflammatory cells or glucose-metabolizing tissues. Understanding the subcellular localization of this compound is essential for optimizing its therapeutic applications.
Preparation Methods
Synthetic Routes and Reaction Conditions
Batatasin I can be isolated from the dichloromethane fraction of Dioscorea batatas . The isolation process involves repeated normal-phase silica gel column chromatographies
Chemical Reactions Analysis
Types of Reactions
Batatasin I undergoes various chemical reactions, including:
Oxidation: this compound can be oxidized to form different phenanthrene derivatives.
Reduction: Reduction reactions can modify the phenanthrene structure.
Substitution: Substitution reactions can introduce different functional groups into the phenanthrene ring.
Common Reagents and Conditions
Common reagents used in these reactions include oxidizing agents like potassium permanganate and reducing agents like sodium borohydride. The reactions typically occur under controlled temperature and pH conditions.
Major Products
The major products formed from these reactions are various phenanthrene derivatives with modified functional groups, which can exhibit different biological activities .
Scientific Research Applications
Chemistry: Batatasin I serves as a precursor for synthesizing other phenanthrene derivatives.
Medicine: This compound exhibits anti-inflammatory properties by inhibiting the generation of prostaglandin D2 and leukotriene C4.
Comparison with Similar Compounds
Similar compounds include Batatasin III, Batatasin IV, and Batatasin V . These compounds share a similar phenanthrene structure but differ in their functional groups and biological activities. Batatasin I is unique due to its potent anti-inflammatory and anti-microbial properties .
Properties
IUPAC Name |
2,5,7-trimethoxyphenanthren-3-ol | |
---|---|---|
Source | PubChem | |
URL | https://pubchem.ncbi.nlm.nih.gov | |
Description | Data deposited in or computed by PubChem | |
InChI |
InChI=1S/C17H16O4/c1-19-12-6-11-5-4-10-7-15(20-2)14(18)9-13(10)17(11)16(8-12)21-3/h4-9,18H,1-3H3 | |
Source | PubChem | |
URL | https://pubchem.ncbi.nlm.nih.gov | |
Description | Data deposited in or computed by PubChem | |
InChI Key |
KGYHMWVRKYFQQR-UHFFFAOYSA-N | |
Source | PubChem | |
URL | https://pubchem.ncbi.nlm.nih.gov | |
Description | Data deposited in or computed by PubChem | |
Canonical SMILES |
COC1=CC(=C2C(=C1)C=CC3=CC(=C(C=C32)O)OC)OC | |
Source | PubChem | |
URL | https://pubchem.ncbi.nlm.nih.gov | |
Description | Data deposited in or computed by PubChem | |
Molecular Formula |
C17H16O4 | |
Source | PubChem | |
URL | https://pubchem.ncbi.nlm.nih.gov | |
Description | Data deposited in or computed by PubChem | |
DSSTOX Substance ID |
DTXSID00199403 | |
Record name | 3-Phenanthrenol, 2,5,7-trimethoxy- | |
Source | EPA DSSTox | |
URL | https://comptox.epa.gov/dashboard/DTXSID00199403 | |
Description | DSSTox provides a high quality public chemistry resource for supporting improved predictive toxicology. | |
Molecular Weight |
284.31 g/mol | |
Source | PubChem | |
URL | https://pubchem.ncbi.nlm.nih.gov | |
Description | Data deposited in or computed by PubChem | |
Physical Description |
Solid | |
Record name | Batatasin I | |
Source | Human Metabolome Database (HMDB) | |
URL | http://www.hmdb.ca/metabolites/HMDB0038778 | |
Description | The Human Metabolome Database (HMDB) is a freely available electronic database containing detailed information about small molecule metabolites found in the human body. | |
Explanation | HMDB is offered to the public as a freely available resource. Use and re-distribution of the data, in whole or in part, for commercial purposes requires explicit permission of the authors and explicit acknowledgment of the source material (HMDB) and the original publication (see the HMDB citing page). We ask that users who download significant portions of the database cite the HMDB paper in any resulting publications. | |
CAS No. |
51415-00-0 | |
Record name | Batatasin I | |
Source | CAS Common Chemistry | |
URL | https://commonchemistry.cas.org/detail?cas_rn=51415-00-0 | |
Description | CAS Common Chemistry is an open community resource for accessing chemical information. Nearly 500,000 chemical substances from CAS REGISTRY cover areas of community interest, including common and frequently regulated chemicals, and those relevant to high school and undergraduate chemistry classes. This chemical information, curated by our expert scientists, is provided in alignment with our mission as a division of the American Chemical Society. | |
Explanation | The data from CAS Common Chemistry is provided under a CC-BY-NC 4.0 license, unless otherwise stated. | |
Record name | Batatasin I | |
Source | ChemIDplus | |
URL | https://pubchem.ncbi.nlm.nih.gov/substance/?source=chemidplus&sourceid=0051415000 | |
Description | ChemIDplus is a free, web search system that provides access to the structure and nomenclature authority files used for the identification of chemical substances cited in National Library of Medicine (NLM) databases, including the TOXNET system. | |
Record name | 3-Phenanthrenol, 2,5,7-trimethoxy- | |
Source | EPA DSSTox | |
URL | https://comptox.epa.gov/dashboard/DTXSID00199403 | |
Description | DSSTox provides a high quality public chemistry resource for supporting improved predictive toxicology. | |
Record name | BATATASIN I | |
Source | FDA Global Substance Registration System (GSRS) | |
URL | https://gsrs.ncats.nih.gov/ginas/app/beta/substances/26K4DP7BUA | |
Description | The FDA Global Substance Registration System (GSRS) enables the efficient and accurate exchange of information on what substances are in regulated products. Instead of relying on names, which vary across regulatory domains, countries, and regions, the GSRS knowledge base makes it possible for substances to be defined by standardized, scientific descriptions. | |
Explanation | Unless otherwise noted, the contents of the FDA website (www.fda.gov), both text and graphics, are not copyrighted. They are in the public domain and may be republished, reprinted and otherwise used freely by anyone without the need to obtain permission from FDA. Credit to the U.S. Food and Drug Administration as the source is appreciated but not required. | |
Record name | Batatasin I | |
Source | Human Metabolome Database (HMDB) | |
URL | http://www.hmdb.ca/metabolites/HMDB0038778 | |
Description | The Human Metabolome Database (HMDB) is a freely available electronic database containing detailed information about small molecule metabolites found in the human body. | |
Explanation | HMDB is offered to the public as a freely available resource. Use and re-distribution of the data, in whole or in part, for commercial purposes requires explicit permission of the authors and explicit acknowledgment of the source material (HMDB) and the original publication (see the HMDB citing page). We ask that users who download significant portions of the database cite the HMDB paper in any resulting publications. | |
Melting Point |
148.5 - 149.6 °C | |
Record name | Batatasin I | |
Source | Human Metabolome Database (HMDB) | |
URL | http://www.hmdb.ca/metabolites/HMDB0038778 | |
Description | The Human Metabolome Database (HMDB) is a freely available electronic database containing detailed information about small molecule metabolites found in the human body. | |
Explanation | HMDB is offered to the public as a freely available resource. Use and re-distribution of the data, in whole or in part, for commercial purposes requires explicit permission of the authors and explicit acknowledgment of the source material (HMDB) and the original publication (see the HMDB citing page). We ask that users who download significant portions of the database cite the HMDB paper in any resulting publications. | |
Retrosynthesis Analysis
AI-Powered Synthesis Planning: Our tool employs the Template_relevance Pistachio, Template_relevance Bkms_metabolic, Template_relevance Pistachio_ringbreaker, Template_relevance Reaxys, Template_relevance Reaxys_biocatalysis model, leveraging a vast database of chemical reactions to predict feasible synthetic routes.
One-Step Synthesis Focus: Specifically designed for one-step synthesis, it provides concise and direct routes for your target compounds, streamlining the synthesis process.
Accurate Predictions: Utilizing the extensive PISTACHIO, BKMS_METABOLIC, PISTACHIO_RINGBREAKER, REAXYS, REAXYS_BIOCATALYSIS database, our tool offers high-accuracy predictions, reflecting the latest in chemical research and data.
Strategy Settings
Precursor scoring | Relevance Heuristic |
---|---|
Min. plausibility | 0.01 |
Model | Template_relevance |
Template Set | Pistachio/Bkms_metabolic/Pistachio_ringbreaker/Reaxys/Reaxys_biocatalysis |
Top-N result to add to graph | 6 |
Feasible Synthetic Routes
A: Batatasin I exerts its anti-inflammatory effects primarily by targeting two key enzymes involved in the inflammatory cascade: cyclooxygenase-2 (COX-2) and 5-lipoxygenase (5-LOX) [].
- It inhibits COX-2-dependent prostaglandin D2 (PGD2) generation in a dose-dependent manner. This decrease in PGD2 production is accompanied by a reduction in COX-2 protein levels [].
- This compound also inhibits the production of 5-LOX-dependent leukotriene C4 (LTC4) in a dose-dependent manner [].
- Additionally, it suppresses mast cell degranulation, further contributing to its anti-inflammatory activity [].
A: this compound is a phenanthrene derivative with the molecular formula C17H16O4 and a molecular weight of 284.31 g/mol [].
A: Research suggests that the hydroxyl group at the C-2′ position of this compound plays a crucial role in its α-glucosidase inhibitory activity []. Modifications at this position significantly impact the potency of inhibition. For instance, Batatasin V, lacking this hydroxyl group, completely loses its inhibitory activity against α-glucosidase [].
A: Yes, this compound has shown promising α-glucosidase inhibitory activity in vitro [, ]. This enzyme plays a critical role in carbohydrate digestion and postprandial blood glucose regulation. By inhibiting α-glucosidase, this compound could potentially help control blood sugar levels, suggesting its potential as an antidiabetic agent [, ].
A: this compound demonstrates anti-adipogenic activity by acting on several key regulators of adipogenesis []:
- It inhibits the expression of peroxisome proliferator-activated receptor γ (PPARγ) and CCAAT/enhancer binding protein α (C/EBPα), two crucial transcription factors involved in adipocyte differentiation [].
- It increases the phosphorylation of AMP-activated protein kinase (p-AMPK), which is known to suppress adipogenesis [].
- It enhances the activity of carnitine palmitoyl transferase-1 (CPT-1), promoting fatty acid oxidation and reducing lipid accumulation [].
A: this compound has been isolated from various species of the Dioscorea genus, commonly known as yams [, , , , , ]. Notably, it is found in the tuberous roots of Dioscorea batatas, Dioscorea opposita, and the rhizomes of Dioscorea dumetorum [, , ]. It has also been identified in Cypripedium smithii [].
A: this compound is often isolated and purified using chromatographic techniques like column chromatography and centrifugal partition chromatography [, , ]. Its identification and quantification are typically achieved through spectroscopic methods, including nuclear magnetic resonance (NMR) spectroscopy and high-performance liquid chromatography coupled with mass spectrometry (HPLC-MS) [, , ].
A: Research has explored the use of this compound as a potential corrosion inhibitor []. Studies suggest that it, along with other components extracted from Chinese yam peel, can synergistically protect copper from corrosion in artificial seawater [].
A: Batatasins, including this compound, are considered dormancy-inducing substances in yam bulbils []. Their levels fluctuate throughout the year, potentially influencing the sprouting behavior of yam tubers [, ].
A: Studies have shown that gibberellic acid (GA₃) treatment can increase Batatasin III content in dormant yam tubers []. Conversely, gibberellin biosynthesis inhibitors like ancymidol and mepiquat chloride can lead to a decrease in Batatasin III and V levels while increasing this compound content [].
A: While research on the antifungal activity of this compound is limited, one study indicated its potential antifungal properties along with dioscin, another compound isolated from the Dioscorea species [].
A: this compound, alongside other compounds like ginsenosides, pachymic acid, and diosgenin, contributes to the pharmacological effects of Shenling Baizhu San, a traditional Chinese medicine formula []. This formula is used for replenishing Qi, invigorating the spleen, dispelling dampness, and relieving diarrhea. This compound and other constituents are believed to contribute to its various therapeutic effects [].
Disclaimer and Information on In-Vitro Research Products
Please be aware that all articles and product information presented on BenchChem are intended solely for informational purposes. The products available for purchase on BenchChem are specifically designed for in-vitro studies, which are conducted outside of living organisms. In-vitro studies, derived from the Latin term "in glass," involve experiments performed in controlled laboratory settings using cells or tissues. It is important to note that these products are not categorized as medicines or drugs, and they have not received approval from the FDA for the prevention, treatment, or cure of any medical condition, ailment, or disease. We must emphasize that any form of bodily introduction of these products into humans or animals is strictly prohibited by law. It is essential to adhere to these guidelines to ensure compliance with legal and ethical standards in research and experimentation.