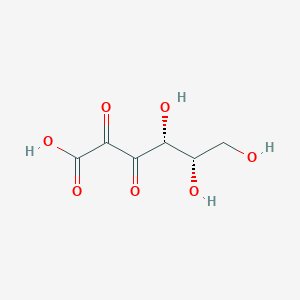
2,3-Diketo-L-gulonic acid
Overview
Description
2,3-Diketo-L-gulonic acid is an organic compound with the molecular formula C6H8O7. It is a carbohydrate acid derived from gulonic acid by the oxidation of the hydroxyl groups at positions 2 and 3 to keto groups . This compound is a key intermediate in the oxidative degradation of L-ascorbic acid (vitamin C) and plays a significant role in various biochemical processes .
Mechanism of Action
Target of Action
2,3-Diketogulonic acid (DKG) is a key metabolite during the degradation of ascorbic acid (Vitamin C) and has been found to have significant impacts on various biological processes .
Mode of Action
The exact mechanism of action of DKG is still under investigation. It’s known that dkg can influence the process of somatic cell reprogramming . It facilitates this process by promoting cell proliferation and inducing the conversion from pre-induced pluripotent stem cells (pre-iPSCs) to iPSCs .
Biochemical Pathways
DKG affects multiple biochemical pathways. It has been found to trigger the mesenchymal-epithelial transition (MET) and activate glycolysis . Notably, DKG alone can activate a non-canonical tricarboxylic acid cycle characterized by increased succinate, fumarate, and malate . This shift redirects oxidative phosphorylation toward glycolysis and induces MET .
Pharmacokinetics
It’s known that dkg is a metabolite in the degradation process of ascorbic acid . After hydrolysis, dehydroascorbic acid (DHA) is irreversibly degraded to DKG, and the half-life of DHA is only minutes due to hydrolytic ring rupture .
Result of Action
The action of DKG results in significant cellular effects. It promotes cell proliferation and induces the conversion from pre-induced pluripotent stem cells (pre-iPSCs) to iPSCs . Moreover, DKG can redirect oxidative phosphorylation toward glycolysis and induce MET , which is a critical process in cellular differentiation and development.
Action Environment
As a metabolite of ascorbic acid, the presence and concentration of DKG can be influenced by the availability and oxidation state of ascorbic acid
Biochemical Analysis
Biochemical Properties
2,3-Diketogulonic acid plays a significant role in biochemical reactions, particularly as a metabolite in the degradation pathway of ascorbic acid (vitamin C). It interacts with several enzymes and proteins during its metabolic conversion. One of the key enzymes involved is 2,3-diketogulonate reductase, which catalyzes the reduction of 2,3-diketogulonic acid to 2-keto-L-gulonic acid. This interaction is crucial for maintaining the balance of ascorbic acid levels in organisms .
Cellular Effects
2,3-Diketogulonic acid influences various cellular processes, including cell signaling pathways, gene expression, and cellular metabolism. In particular, it has been observed to affect the oxidative stress response in cells. By modulating the levels of reactive oxygen species, 2,3-diketogulonic acid can impact cell survival and apoptosis. Additionally, it has been shown to influence the expression of genes involved in antioxidant defense mechanisms, thereby playing a role in cellular protection against oxidative damage .
Molecular Mechanism
At the molecular level, 2,3-diketogulonic acid exerts its effects through several mechanisms. It can bind to specific biomolecules, such as enzymes and proteins, altering their activity. For example, 2,3-diketogulonic acid can inhibit the activity of certain enzymes involved in the ascorbic acid degradation pathway, leading to changes in the levels of ascorbic acid and its metabolites. Additionally, it can modulate gene expression by influencing transcription factors and other regulatory proteins, thereby affecting cellular functions .
Temporal Effects in Laboratory Settings
In laboratory settings, the effects of 2,3-diketogulonic acid can change over time due to its stability and degradation. Studies have shown that 2,3-diketogulonic acid is relatively stable under certain conditions but can degrade over time, leading to the formation of other metabolites. Long-term exposure to 2,3-diketogulonic acid has been observed to cause changes in cellular function, including alterations in metabolic pathways and gene expression .
Dosage Effects in Animal Models
The effects of 2,3-diketogulonic acid vary with different dosages in animal models. At low doses, it may have minimal impact on cellular functions, while higher doses can lead to significant changes in metabolic pathways and cellular processes. Threshold effects have been observed, where a certain dosage level is required to elicit a noticeable response. Additionally, high doses of 2,3-diketogulonic acid can result in toxic or adverse effects, including oxidative stress and cellular damage .
Metabolic Pathways
2,3-Diketogulonic acid is involved in several metabolic pathways, including the degradation of ascorbic acid. It interacts with enzymes such as 2,3-diketogulonate reductase and other cofactors that facilitate its conversion to other metabolites. These interactions can affect metabolic flux and the levels of various metabolites within the cell. Understanding the metabolic pathways involving 2,3-diketogulonic acid is crucial for elucidating its role in cellular metabolism .
Transport and Distribution
Within cells and tissues, 2,3-diketogulonic acid is transported and distributed through specific transporters and binding proteins. These interactions can influence its localization and accumulation in different cellular compartments. For example, certain transporters may facilitate the uptake of 2,3-diketogulonic acid into mitochondria, where it can participate in metabolic reactions. The distribution of 2,3-diketogulonic acid within cells is essential for its proper function and regulation .
Subcellular Localization
The subcellular localization of 2,3-diketogulonic acid can affect its activity and function. It may be directed to specific compartments or organelles through targeting signals or post-translational modifications. For instance, 2,3-diketogulonic acid may localize to the mitochondria, where it can influence mitochondrial metabolism and oxidative stress responses. Understanding the subcellular localization of 2,3-diketogulonic acid is important for elucidating its role in cellular processes .
Preparation Methods
Synthetic Routes and Reaction Conditions: 2,3-Diketo-L-gulonic acid can be synthesized through the oxidation of L-ascorbic acid. The process involves the initial oxidation of L-ascorbic acid to dehydroascorbic acid, which then undergoes further oxidation to form this compound . The reaction conditions typically include the presence of oxidizing agents such as oxygen or metal ions, and the process can be influenced by factors like pH and temperature .
Industrial Production Methods: Industrial production of this compound often involves the controlled oxidation of L-ascorbic acid using specific catalysts and reaction conditions to ensure high yield and purity. The process may also include steps to isolate and purify the compound from the reaction mixture .
Chemical Reactions Analysis
Types of Reactions: 2,3-Diketo-L-gulonic acid undergoes various chemical reactions, including:
Oxidation: Further oxidation of this compound can lead to the formation of oxalic acid and other degradation products.
Reduction: It can be reduced back to L-ascorbic acid under specific conditions.
Hydrolysis: Dehydroascorbic acid can undergo irreversible hydrolysis to form this compound.
Common Reagents and Conditions:
Oxidizing Agents: Oxygen, metal ions (e.g., copper, iron).
Reducing Agents: Hydrogen, metal hydrides.
Hydrolysis Conditions: Acidic or basic conditions, elevated temperatures.
Major Products Formed:
Oxalic Acid: A common degradation product formed through further oxidation.
L-Ascorbic Acid: Formed through reduction reactions.
Scientific Research Applications
2,3-Diketo-L-gulonic acid has several scientific research applications, including:
Comparison with Similar Compounds
L-Ascorbic Acid (Vitamin C): A precursor to 2,3-Diketo-L-gulonic acid, known for its antioxidant properties.
Dehydroascorbic Acid: An intermediate in the oxidation of L-ascorbic acid, which can be hydrolyzed to form this compound.
Oxalic Acid: A degradation product formed through the further oxidation of this compound.
Uniqueness: this compound is unique due to its role as a key intermediate in the oxidative degradation of L-ascorbic acid. Its ability to participate in various biochemical pathways and its potential antioxidant properties make it a compound of significant interest in scientific research .
Properties
IUPAC Name |
(4R,5S)-4,5,6-trihydroxy-2,3-dioxohexanoic acid | |
---|---|---|
Source | PubChem | |
URL | https://pubchem.ncbi.nlm.nih.gov | |
Description | Data deposited in or computed by PubChem | |
InChI |
InChI=1S/C6H8O7/c7-1-2(8)3(9)4(10)5(11)6(12)13/h2-3,7-9H,1H2,(H,12,13)/t2-,3+/m0/s1 | |
Source | PubChem | |
URL | https://pubchem.ncbi.nlm.nih.gov | |
Description | Data deposited in or computed by PubChem | |
InChI Key |
GJQWCDSAOUMKSE-STHAYSLISA-N | |
Source | PubChem | |
URL | https://pubchem.ncbi.nlm.nih.gov | |
Description | Data deposited in or computed by PubChem | |
Canonical SMILES |
C(C(C(C(=O)C(=O)C(=O)O)O)O)O | |
Source | PubChem | |
URL | https://pubchem.ncbi.nlm.nih.gov | |
Description | Data deposited in or computed by PubChem | |
Isomeric SMILES |
C([C@@H]([C@H](C(=O)C(=O)C(=O)O)O)O)O | |
Source | PubChem | |
URL | https://pubchem.ncbi.nlm.nih.gov | |
Description | Data deposited in or computed by PubChem | |
Molecular Formula |
C6H8O7 | |
Source | PubChem | |
URL | https://pubchem.ncbi.nlm.nih.gov | |
Description | Data deposited in or computed by PubChem | |
DSSTOX Substance ID |
DTXSID40331486 | |
Record name | 2,3-diketo-L-gulonate | |
Source | EPA DSSTox | |
URL | https://comptox.epa.gov/dashboard/DTXSID40331486 | |
Description | DSSTox provides a high quality public chemistry resource for supporting improved predictive toxicology. | |
Molecular Weight |
192.12 g/mol | |
Source | PubChem | |
URL | https://pubchem.ncbi.nlm.nih.gov | |
Description | Data deposited in or computed by PubChem | |
Physical Description |
Solid | |
Record name | Diketogulonic acid | |
Source | Human Metabolome Database (HMDB) | |
URL | http://www.hmdb.ca/metabolites/HMDB0005971 | |
Description | The Human Metabolome Database (HMDB) is a freely available electronic database containing detailed information about small molecule metabolites found in the human body. | |
Explanation | HMDB is offered to the public as a freely available resource. Use and re-distribution of the data, in whole or in part, for commercial purposes requires explicit permission of the authors and explicit acknowledgment of the source material (HMDB) and the original publication (see the HMDB citing page). We ask that users who download significant portions of the database cite the HMDB paper in any resulting publications. | |
CAS No. |
3445-22-5, 3409-57-2 | |
Record name | L-threo-2,3-Hexodiulosonic acid | |
Source | CAS Common Chemistry | |
URL | https://commonchemistry.cas.org/detail?cas_rn=3445-22-5 | |
Description | CAS Common Chemistry is an open community resource for accessing chemical information. Nearly 500,000 chemical substances from CAS REGISTRY cover areas of community interest, including common and frequently regulated chemicals, and those relevant to high school and undergraduate chemistry classes. This chemical information, curated by our expert scientists, is provided in alignment with our mission as a division of the American Chemical Society. | |
Explanation | The data from CAS Common Chemistry is provided under a CC-BY-NC 4.0 license, unless otherwise stated. | |
Record name | 2,3-diketo-L-gulonate | |
Source | EPA DSSTox | |
URL | https://comptox.epa.gov/dashboard/DTXSID40331486 | |
Description | DSSTox provides a high quality public chemistry resource for supporting improved predictive toxicology. | |
Record name | Diketogulonic acid | |
Source | Human Metabolome Database (HMDB) | |
URL | http://www.hmdb.ca/metabolites/HMDB0005971 | |
Description | The Human Metabolome Database (HMDB) is a freely available electronic database containing detailed information about small molecule metabolites found in the human body. | |
Explanation | HMDB is offered to the public as a freely available resource. Use and re-distribution of the data, in whole or in part, for commercial purposes requires explicit permission of the authors and explicit acknowledgment of the source material (HMDB) and the original publication (see the HMDB citing page). We ask that users who download significant portions of the database cite the HMDB paper in any resulting publications. | |
Retrosynthesis Analysis
AI-Powered Synthesis Planning: Our tool employs the Template_relevance Pistachio, Template_relevance Bkms_metabolic, Template_relevance Pistachio_ringbreaker, Template_relevance Reaxys, Template_relevance Reaxys_biocatalysis model, leveraging a vast database of chemical reactions to predict feasible synthetic routes.
One-Step Synthesis Focus: Specifically designed for one-step synthesis, it provides concise and direct routes for your target compounds, streamlining the synthesis process.
Accurate Predictions: Utilizing the extensive PISTACHIO, BKMS_METABOLIC, PISTACHIO_RINGBREAKER, REAXYS, REAXYS_BIOCATALYSIS database, our tool offers high-accuracy predictions, reflecting the latest in chemical research and data.
Strategy Settings
Precursor scoring | Relevance Heuristic |
---|---|
Min. plausibility | 0.01 |
Model | Template_relevance |
Template Set | Pistachio/Bkms_metabolic/Pistachio_ringbreaker/Reaxys/Reaxys_biocatalysis |
Top-N result to add to graph | 6 |
Feasible Synthetic Routes
Q1: What is the primary pathway of 2,3-diketogulonic acid formation?
A1: 2,3-Diketogulonic acid (2,3-DKG) primarily forms through the irreversible hydrolysis of dehydroascorbic acid (DHA), which is the oxidized form of L-ascorbic acid (vitamin C). [, ]
Q2: What factors influence the degradation of vitamin C into 2,3-DKG?
A2: Several factors impact the degradation rate, including:
- pH: 2,3-DKG formation is favored at pH values above 7. [, ]
- Temperature: Heat accelerates ascorbic acid oxidation and subsequent 2,3-DKG formation. [, ]
- Oxygen Presence: The presence of oxygen promotes the oxidation of ascorbic acid to DHA, a precursor to 2,3-DKG. [, ]
- Light Exposure: Ultraviolet (UV) light, specifically UV-C at 254 nm, has been shown to induce ascorbic acid degradation, leading to increased levels of DHA and 2,3-DKG. []
- Metal Ions: Trace metals like copper can catalyze ascorbic acid oxidation, contributing to 2,3-DKG formation. [, ]
Q3: Does 2,3-diketogulonic acid further degrade?
A3: Yes, 2,3-DKG can further degrade, particularly at high pH values. This degradation can lead to the formation of other compounds like threonic acid, xylonic acid, and oxalic acid. []
Q4: What is the role of 2,3-diketogulonic acid in glycation?
A4: While 2,3-DKG itself is not a strong glycating agent, its precursor, DHA, can contribute to the formation of advanced glycation end products (AGEs) through the Maillard reaction. [, ]
Q5: How does high galactose impact ascorbate metabolism and glycation?
A5: Research indicates that high galactose levels can disrupt the balance between ascorbic acid and DHA, leading to increased 2,3-DKG production in rat lenses. This disruption, coupled with potential changes in lens permeability to 2,3-DKG, may contribute to increased AGE formation. []
Q6: What evidence suggests that ascorbic acid can act as a precursor for AGEs?
A6: Studies show that incubating bovine lens crystallins or polylysine with ascorbic acid under reducing conditions leads to the formation of Nε-(carboxymethyl)lysine (CML), a major AGE. This reaction is also observed with DHA. []
Q7: Does the presence of sodium cyanoborohydride (NaCNBH3) influence CML formation?
A7: Yes, NaCNBH3 plays a crucial role. In its presence, CML formation is significantly enhanced when ascorbic acid or DHA are used. This suggests that NaCNBH3 might be reducing a Schiff base intermediate formed between lysine and DHA, leading to CML. []
Q8: What analytical techniques are used to study 2,3-DKG?
A8: Various techniques are employed for the analysis of 2,3-DKG and related compounds, including:
- High-Performance Liquid Chromatography (HPLC): Often coupled with UV detection or mass spectrometry (MS), HPLC is crucial for separating and quantifying 2,3-DKG in complex mixtures like food products or biological samples. [, ]
- Mass Spectrometry (MS): MS techniques, such as LC-MS/MS, provide high sensitivity and selectivity, enabling the identification and quantification of 2,3-DKG and other related compounds in biological matrices like blood plasma. [, ]
- Spectrophotometry: This technique utilizes the absorbance properties of 2,3-DKG and related compounds for their quantification, particularly in biological samples. [, ]
- Electron Spin Resonance (ESR) Spectroscopy: This technique helps in understanding the mechanism of ascorbic acid degradation by detecting the formation of free radicals, such as the ascorbyl radical. []
- Gas Chromatography-Mass Spectrometry (GC-MS): This technique can be used to study the kinetics of ascorbic acid and DHA redox reactions in complex matrices like wheat dough. []
Q9: Are there specific challenges in analyzing 2,3-DKG?
A9: Yes, the instability of 2,3-DKG poses a significant challenge. It is prone to further degradation, making accurate quantification difficult. Researchers often employ specific extraction techniques and work quickly to minimize degradation during analysis. []
Disclaimer and Information on In-Vitro Research Products
Please be aware that all articles and product information presented on BenchChem are intended solely for informational purposes. The products available for purchase on BenchChem are specifically designed for in-vitro studies, which are conducted outside of living organisms. In-vitro studies, derived from the Latin term "in glass," involve experiments performed in controlled laboratory settings using cells or tissues. It is important to note that these products are not categorized as medicines or drugs, and they have not received approval from the FDA for the prevention, treatment, or cure of any medical condition, ailment, or disease. We must emphasize that any form of bodily introduction of these products into humans or animals is strictly prohibited by law. It is essential to adhere to these guidelines to ensure compliance with legal and ethical standards in research and experimentation.