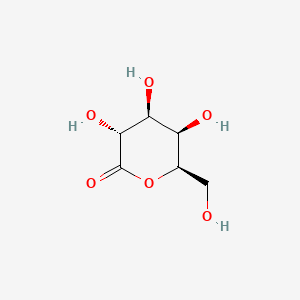
D-galactonolactone
- Click on QUICK INQUIRY to receive a quote from our team of experts.
- With the quality product at a COMPETITIVE price, you can focus more on your research.
Overview
Description
D-Galactopyranosyl-1-On is a derivative of galactose, a monosaccharide sugar that is a constituent of lactose. This compound is significant in various biochemical processes and has applications in multiple fields, including food science, pharmaceuticals, and biotechnology. Its structure consists of a galactose molecule in its pyranose form, which is a six-membered ring with five carbon atoms and one oxygen atom.
Preparation Methods
Synthetic Routes and Reaction Conditions: The synthesis of D-Galactopyranosyl-1-On typically involves the oxidation of D-galactose. One common method is the use of galactose oxidase, an enzyme that specifically oxidizes the primary alcohol group of D-galactose to form the corresponding aldehyde, D-Galactopyranosyl-1-On . This reaction is usually carried out in an aqueous buffer solution at a pH of around 7.0 and at room temperature.
Industrial Production Methods: In an industrial setting, the production of D-Galactopyranosyl-1-On can be scaled up using bioreactors where galactose oxidase is immobilized on a solid support. This allows for continuous production and easy separation of the enzyme from the product. The reaction conditions are optimized to ensure maximum yield and purity of the product .
Chemical Reactions Analysis
Types of Reactions: D-Galactopyranosyl-1-On undergoes several types of chemical reactions, including:
Oxidation: Further oxidation can convert the aldehyde group to a carboxylic acid.
Reduction: The aldehyde group can be reduced to a primary alcohol.
Substitution: The aldehyde group can participate in nucleophilic substitution reactions to form various derivatives.
Common Reagents and Conditions:
Oxidation: Reagents such as potassium permanganate or nitric acid can be used under acidic conditions.
Reduction: Sodium borohydride or lithium aluminum hydride are common reducing agents used under mild conditions.
Major Products:
Oxidation: Galacturonic acid.
Reduction: D-Galactopyranosyl alcohol.
Substitution: Various imines and thioethers depending on the nucleophile used.
Scientific Research Applications
D-Galactopyranosyl-1-On has a wide range of applications in scientific research:
Chemistry: It is used as a building block in the synthesis of more complex carbohydrates and glycoconjugates.
Biology: It serves as a substrate for studying the activity of galactose oxidase and other related enzymes.
Medicine: It is used in the development of diagnostic assays for detecting galactose metabolism disorders.
Industry: It is employed in the production of bio-based materials and as a precursor for the synthesis of biodegradable polymers
Mechanism of Action
The primary mechanism of action of D-Galactopyranosyl-1-On involves its role as a substrate for various enzymes. For example, galactose oxidase catalyzes the oxidation of D-Galactopyranosyl-1-On to produce hydrogen peroxide and D-Galactopyranosyl acid. This reaction is crucial in various metabolic pathways and can be used to generate reactive oxygen species for studying oxidative stress in cells .
Comparison with Similar Compounds
D-Glucopyranosyl-1-On: Similar in structure but derived from glucose.
D-Mannopyranosyl-1-On: Derived from mannose and has similar chemical properties.
Uniqueness: D-Galactopyranosyl-1-On is unique due to its specific role in galactose metabolism and its ability to form stable derivatives through various chemical reactions. Its applications in biotechnology and medicine also distinguish it from other similar compounds .
Biological Activity
D-Galactonolactone is a cyclic ester derived from D-galactose, playing a significant role in various biological processes. Its primary relevance is observed in the metabolic pathways involving galactose, particularly in its conversion to ascorbic acid (vitamin C) and its implications in galactose metabolism disorders. This article explores the biological activity of this compound, including its enzymatic interactions, metabolic pathways, and clinical significance.
Metabolic Pathway
This compound is produced through the oxidation of D-galactose by the enzyme NAD+-dependent galactose dehydrogenase (EC 1.1.1.48). This reaction is crucial in the metabolic pathway that leads to the synthesis of ascorbic acid:
The subsequent hydrolysis of this compound by lactonase results in D-galactonate, which can enter various metabolic pathways, including the Krebs cycle for energy production .
Galactonolactone Dehydrogenase (GALDH)
GALDH plays a pivotal role in the conversion of this compound to L-ascorbic acid. Studies have shown that GALDH activity is sensitive to oxidative stress, which can lead to enzyme inactivation. The presence of this compound has been found to protect GALDH from thiol modification, thereby enhancing its stability and activity under oxidative conditions .
Table 1: GALDH Activity Under Different Conditions
Treatment | Relative Activity (%) | Modification Type |
---|---|---|
Control | 100 | None |
H₂O₂ (1 h) | 24 | Sulfinic acid |
H₂O₂ (3 h) | 1.4 | Sulfonic acid |
H₂O₂ + DTT (3 h) | 6.9 | Not determined |
H₂O₂/GSH | 0.3 | Glutathionylation |
This table illustrates how oxidative stress affects GALDH activity, highlighting the protective role of this compound .
Galactosemia
This compound's significance is particularly evident in patients with galactosemia , a genetic disorder affecting galactose metabolism due to deficiencies in specific enzymes such as galactose-1-phosphate uridylyltransferase (GALT). In these patients, elevated levels of this compound and its metabolites, such as galactonate, are observed. Research indicates that individuals with GALT deficiency excrete significantly higher amounts of galactonate when subjected to a galactose load compared to healthy subjects .
Case Study: Urinary Excretion of Galactonate
A study involving infants and adults with GALT deficiency revealed that they excreted large amounts of urinary galactonate, which decreased upon implementing a lactose-free diet. This finding underscores the importance of monitoring this compound and related metabolites in managing galactosemia .
Properties
CAS No. |
15892-28-1 |
---|---|
Molecular Formula |
C6H10O6 |
Molecular Weight |
178.14 g/mol |
IUPAC Name |
(3R,4S,5R,6R)-3,4,5-trihydroxy-6-(hydroxymethyl)oxan-2-one |
InChI |
InChI=1S/C6H10O6/c7-1-2-3(8)4(9)5(10)6(11)12-2/h2-5,7-10H,1H2/t2-,3+,4+,5-/m1/s1 |
InChI Key |
PHOQVHQSTUBQQK-MGCNEYSASA-N |
SMILES |
C(C1C(C(C(C(=O)O1)O)O)O)O |
Isomeric SMILES |
C([C@@H]1[C@@H]([C@@H]([C@H](C(=O)O1)O)O)O)O |
Canonical SMILES |
C(C1C(C(C(C(=O)O1)O)O)O)O |
Origin of Product |
United States |
Retrosynthesis Analysis
AI-Powered Synthesis Planning: Our tool employs the Template_relevance Pistachio, Template_relevance Bkms_metabolic, Template_relevance Pistachio_ringbreaker, Template_relevance Reaxys, Template_relevance Reaxys_biocatalysis model, leveraging a vast database of chemical reactions to predict feasible synthetic routes.
One-Step Synthesis Focus: Specifically designed for one-step synthesis, it provides concise and direct routes for your target compounds, streamlining the synthesis process.
Accurate Predictions: Utilizing the extensive PISTACHIO, BKMS_METABOLIC, PISTACHIO_RINGBREAKER, REAXYS, REAXYS_BIOCATALYSIS database, our tool offers high-accuracy predictions, reflecting the latest in chemical research and data.
Strategy Settings
Precursor scoring | Relevance Heuristic |
---|---|
Min. plausibility | 0.01 |
Model | Template_relevance |
Template Set | Pistachio/Bkms_metabolic/Pistachio_ringbreaker/Reaxys/Reaxys_biocatalysis |
Top-N result to add to graph | 6 |
Feasible Synthetic Routes
Disclaimer and Information on In-Vitro Research Products
Please be aware that all articles and product information presented on BenchChem are intended solely for informational purposes. The products available for purchase on BenchChem are specifically designed for in-vitro studies, which are conducted outside of living organisms. In-vitro studies, derived from the Latin term "in glass," involve experiments performed in controlled laboratory settings using cells or tissues. It is important to note that these products are not categorized as medicines or drugs, and they have not received approval from the FDA for the prevention, treatment, or cure of any medical condition, ailment, or disease. We must emphasize that any form of bodily introduction of these products into humans or animals is strictly prohibited by law. It is essential to adhere to these guidelines to ensure compliance with legal and ethical standards in research and experimentation.