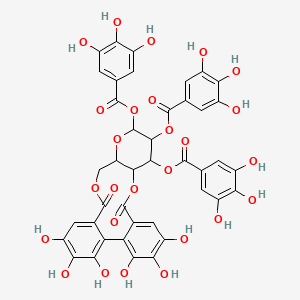
Tellimagrandin II
Overview
Description
Tellimagrandin II is a type of ellagitannin, a class of hydrolyzable tannins. It is the first of the ellagitannins formed from 1,2,3,4,6-pentagalloyl-glucose. This compound can be found in plants such as Geum japonicum and Syzygium aromaticum (clove) . This compound is known for its various biological activities, including anti-herpesvirus properties .
Preparation Methods
Tellimagrandin II is formed by the oxidation of pentagalloyl glucose in Tellima grandiflora by the enzyme pentagalloylglucose: O (2) oxidoreductase, a laccase-type phenol oxidase . This compound can also be extracted from the shells of Trapa bispinosa . Industrial production methods typically involve the extraction and purification of the compound from natural sources, followed by various chromatographic techniques to isolate the pure compound.
Chemical Reactions Analysis
Tellimagrandin II undergoes several types of chemical reactions:
Oxidation: It is formed by the oxidation of pentagalloyl glucose.
Dimerization: It can be dimerized to form cornusiin E in the presence of laccase.
Hydrolysis: The compound can be hydrolyzed to yield gallic acid and glucose derivatives.
Common reagents and conditions used in these reactions include oxidizing agents like laccase and various hydrolytic enzymes. The major products formed from these reactions include gallic acid, glucose derivatives, and dimerized compounds like cornusiin E .
Scientific Research Applications
Tellimagrandin II has a wide range of scientific research applications:
Chemistry: It is studied for its unique chemical structure and reactivity.
Medicine: This compound has shown potential as an anti-herpesvirus agent.
Industry: The compound is used in the development of natural preservatives and antimicrobial agents.
Mechanism of Action
Tellimagrandin II exerts its effects through several mechanisms:
Antibacterial Activity: It reduces the expression of mecA, followed by the negative regulation of the penicillin-binding protein 2a (PBP2a) of MRSA.
Antiviral Activity: The compound shows anti-herpesvirus properties, although the exact molecular targets and pathways involved are still under investigation.
Comparison with Similar Compounds
Tellimagrandin II is similar to other ellagitannins such as punicafolin and nupharin A, but it is unique in that the hexahydroxydiphenoyl group is not attached to the same hydroxyl groups in the glucose molecule . Other similar compounds include:
Tellimagrandin I: Differs from Tellimagrandin II by a hydroxyl group instead of a third galloyl group.
Punigluconin: Another ellagitannin monomer similar in structure.
Pedunculagin: Structurally similar to Tellimagrandin II.
Tellimagrandin II stands out due to its specific biological activities and unique structural features.
Properties
IUPAC Name |
[3,4,5,21,22,23-hexahydroxy-8,18-dioxo-12,13-bis[(3,4,5-trihydroxybenzoyl)oxy]-9,14,17-trioxatetracyclo[17.4.0.02,7.010,15]tricosa-1(23),2,4,6,19,21-hexaen-11-yl] 3,4,5-trihydroxybenzoate | |
---|---|---|
Details | Computed by Lexichem TK 2.7.0 (PubChem release 2021.05.07) | |
Source | PubChem | |
URL | https://pubchem.ncbi.nlm.nih.gov | |
Description | Data deposited in or computed by PubChem | |
InChI |
InChI=1S/C41H30O26/c42-15-1-10(2-16(43)26(15)50)36(57)65-34-33-23(9-62-39(60)13-7-21(48)29(53)31(55)24(13)25-14(40(61)64-33)8-22(49)30(54)32(25)56)63-41(67-38(59)12-5-19(46)28(52)20(47)6-12)35(34)66-37(58)11-3-17(44)27(51)18(45)4-11/h1-8,23,33-35,41-56H,9H2 | |
Details | Computed by InChI 1.0.6 (PubChem release 2021.05.07) | |
Source | PubChem | |
URL | https://pubchem.ncbi.nlm.nih.gov | |
Description | Data deposited in or computed by PubChem | |
InChI Key |
JCGHAEBIBSEQAD-UHFFFAOYSA-N | |
Details | Computed by InChI 1.0.6 (PubChem release 2021.05.07) | |
Source | PubChem | |
URL | https://pubchem.ncbi.nlm.nih.gov | |
Description | Data deposited in or computed by PubChem | |
Canonical SMILES |
C1C2C(C(C(C(O2)OC(=O)C3=CC(=C(C(=C3)O)O)O)OC(=O)C4=CC(=C(C(=C4)O)O)O)OC(=O)C5=CC(=C(C(=C5)O)O)O)OC(=O)C6=CC(=C(C(=C6C7=C(C(=C(C=C7C(=O)O1)O)O)O)O)O)O | |
Details | Computed by OEChem 2.3.0 (PubChem release 2021.05.07) | |
Source | PubChem | |
URL | https://pubchem.ncbi.nlm.nih.gov | |
Description | Data deposited in or computed by PubChem | |
Molecular Formula |
C41H30O26 | |
Details | Computed by PubChem 2.1 (PubChem release 2021.05.07) | |
Source | PubChem | |
URL | https://pubchem.ncbi.nlm.nih.gov | |
Description | Data deposited in or computed by PubChem | |
DSSTOX Substance ID |
DTXSID201002061 | |
Record name | Tellimagrandin II | |
Source | EPA DSSTox | |
URL | https://comptox.epa.gov/dashboard/DTXSID201002061 | |
Description | DSSTox provides a high quality public chemistry resource for supporting improved predictive toxicology. | |
Molecular Weight |
938.7 g/mol | |
Details | Computed by PubChem 2.1 (PubChem release 2021.05.07) | |
Source | PubChem | |
URL | https://pubchem.ncbi.nlm.nih.gov | |
Description | Data deposited in or computed by PubChem | |
CAS No. |
81571-72-4, 58970-75-5 | |
Record name | Tellimagrandin II | |
Source | CAS Common Chemistry | |
URL | https://commonchemistry.cas.org/detail?cas_rn=81571-72-4 | |
Description | CAS Common Chemistry is an open community resource for accessing chemical information. Nearly 500,000 chemical substances from CAS REGISTRY cover areas of community interest, including common and frequently regulated chemicals, and those relevant to high school and undergraduate chemistry classes. This chemical information, curated by our expert scientists, is provided in alignment with our mission as a division of the American Chemical Society. | |
Explanation | The data from CAS Common Chemistry is provided under a CC-BY-NC 4.0 license, unless otherwise stated. | |
Record name | Eugeniin | |
Source | ChemIDplus | |
URL | https://pubchem.ncbi.nlm.nih.gov/substance/?source=chemidplus&sourceid=0058970755 | |
Description | ChemIDplus is a free, web search system that provides access to the structure and nomenclature authority files used for the identification of chemical substances cited in National Library of Medicine (NLM) databases, including the TOXNET system. | |
Record name | Tellimagrandin II | |
Source | ChemIDplus | |
URL | https://pubchem.ncbi.nlm.nih.gov/substance/?source=chemidplus&sourceid=0081571724 | |
Description | ChemIDplus is a free, web search system that provides access to the structure and nomenclature authority files used for the identification of chemical substances cited in National Library of Medicine (NLM) databases, including the TOXNET system. | |
Record name | Tellimagrandin II | |
Source | EPA DSSTox | |
URL | https://comptox.epa.gov/dashboard/DTXSID201002061 | |
Description | DSSTox provides a high quality public chemistry resource for supporting improved predictive toxicology. | |
Record name | Tellimagrandin II | |
Source | Human Metabolome Database (HMDB) | |
URL | http://www.hmdb.ca/metabolites/HMDB0039265 | |
Description | The Human Metabolome Database (HMDB) is a freely available electronic database containing detailed information about small molecule metabolites found in the human body. | |
Explanation | HMDB is offered to the public as a freely available resource. Use and re-distribution of the data, in whole or in part, for commercial purposes requires explicit permission of the authors and explicit acknowledgment of the source material (HMDB) and the original publication (see the HMDB citing page). We ask that users who download significant portions of the database cite the HMDB paper in any resulting publications. | |
Disclaimer and Information on In-Vitro Research Products
Please be aware that all articles and product information presented on BenchChem are intended solely for informational purposes. The products available for purchase on BenchChem are specifically designed for in-vitro studies, which are conducted outside of living organisms. In-vitro studies, derived from the Latin term "in glass," involve experiments performed in controlled laboratory settings using cells or tissues. It is important to note that these products are not categorized as medicines or drugs, and they have not received approval from the FDA for the prevention, treatment, or cure of any medical condition, ailment, or disease. We must emphasize that any form of bodily introduction of these products into humans or animals is strictly prohibited by law. It is essential to adhere to these guidelines to ensure compliance with legal and ethical standards in research and experimentation.