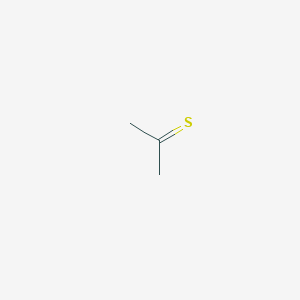
Thioacetone
Overview
Description
Thioacetone is an organosulfur compound belonging to the thioketone group, with the chemical formula (CH₃)₂CS. It is an unstable orange or brown substance that can only be isolated at low temperatures. Above −20°C, this compound readily converts to a polymer and a trimer, trithis compound. It is known for its extremely potent and unpleasant odor, considered one of the worst-smelling chemicals known to humanity .
Preparation Methods
Thioacetone is typically obtained by cracking the cyclic trimer trithis compound, [(CH₃)₂CS]₃. The trimer is prepared by pyrolysis of allyl isopropyl sulfide or by treating acetone with hydrogen sulfide in the presence of a Lewis acid. The trimer cracks at 500–600°C to give the thione .
Chemical Reactions Analysis
Thioacetone undergoes several types of chemical reactions, including:
Reactions with Alkyllithiums: This compound reacts with organolithium reagents to give a variety of products, often without forming a thiol.
Common reagents and conditions used in these reactions include hydrogen sulfide, Lewis acids, and organolithium reagents. Major products formed from these reactions include the polymer and trithis compound .
Scientific Research Applications
Thioacetone has been studied for its interaction with carbon dioxide and water. Research shows that complexes of this compound with carbon dioxide and water are stabilized by C⋯O tetrel bonds, C⋯S bonds, and hydrogen bonds. This study is significant for understanding molecular interactions and could have implications in fields like environmental chemistry and material science.
Mechanism of Action
The mechanism by which thioacetone exerts its effects involves its high reactivity and tendency to polymerize. The molecular targets and pathways involved include interactions with carbon dioxide and water, leading to the formation of stabilized complexes.
Comparison with Similar Compounds
Thioacetone is similar to other thioketones and ketones. Thioketones contain a thionic group (>C=S), which is less polarized but more polarizable than the carbonyl group (>C=O) found in ketones. This difference in molecular structure leads to variations in chemical reactivity and properties. Similar compounds include acetone, 2-butanone, and 2-pentanone .
This compound’s uniqueness lies in its extremely potent odor and its high reactivity, which makes it a subject of interest in various scientific studies .
Properties
IUPAC Name |
propane-2-thione | |
---|---|---|
Source | PubChem | |
URL | https://pubchem.ncbi.nlm.nih.gov | |
Description | Data deposited in or computed by PubChem | |
InChI |
InChI=1S/C3H6S/c1-3(2)4/h1-2H3 | |
Source | PubChem | |
URL | https://pubchem.ncbi.nlm.nih.gov | |
Description | Data deposited in or computed by PubChem | |
InChI Key |
JTNXQVCPQMQLHK-UHFFFAOYSA-N | |
Source | PubChem | |
URL | https://pubchem.ncbi.nlm.nih.gov | |
Description | Data deposited in or computed by PubChem | |
Canonical SMILES |
CC(=S)C | |
Source | PubChem | |
URL | https://pubchem.ncbi.nlm.nih.gov | |
Description | Data deposited in or computed by PubChem | |
Molecular Formula |
C3H6S | |
Source | PubChem | |
URL | https://pubchem.ncbi.nlm.nih.gov | |
Description | Data deposited in or computed by PubChem | |
Molecular Weight |
74.15 g/mol | |
Source | PubChem | |
URL | https://pubchem.ncbi.nlm.nih.gov | |
Description | Data deposited in or computed by PubChem | |
CAS No. |
4756-05-2 | |
Record name | 2-Propanethione | |
Source | CAS Common Chemistry | |
URL | https://commonchemistry.cas.org/detail?cas_rn=4756-05-2 | |
Description | CAS Common Chemistry is an open community resource for accessing chemical information. Nearly 500,000 chemical substances from CAS REGISTRY cover areas of community interest, including common and frequently regulated chemicals, and those relevant to high school and undergraduate chemistry classes. This chemical information, curated by our expert scientists, is provided in alignment with our mission as a division of the American Chemical Society. | |
Explanation | The data from CAS Common Chemistry is provided under a CC-BY-NC 4.0 license, unless otherwise stated. | |
Record name | Thioacetone | |
Source | ChemIDplus | |
URL | https://pubchem.ncbi.nlm.nih.gov/substance/?source=chemidplus&sourceid=0004756052 | |
Description | ChemIDplus is a free, web search system that provides access to the structure and nomenclature authority files used for the identification of chemical substances cited in National Library of Medicine (NLM) databases, including the TOXNET system. | |
Retrosynthesis Analysis
AI-Powered Synthesis Planning: Our tool employs the Template_relevance Pistachio, Template_relevance Bkms_metabolic, Template_relevance Pistachio_ringbreaker, Template_relevance Reaxys, Template_relevance Reaxys_biocatalysis model, leveraging a vast database of chemical reactions to predict feasible synthetic routes.
One-Step Synthesis Focus: Specifically designed for one-step synthesis, it provides concise and direct routes for your target compounds, streamlining the synthesis process.
Accurate Predictions: Utilizing the extensive PISTACHIO, BKMS_METABOLIC, PISTACHIO_RINGBREAKER, REAXYS, REAXYS_BIOCATALYSIS database, our tool offers high-accuracy predictions, reflecting the latest in chemical research and data.
Strategy Settings
Precursor scoring | Relevance Heuristic |
---|---|
Min. plausibility | 0.01 |
Model | Template_relevance |
Template Set | Pistachio/Bkms_metabolic/Pistachio_ringbreaker/Reaxys/Reaxys_biocatalysis |
Top-N result to add to graph | 6 |
Feasible Synthetic Routes
Disclaimer and Information on In-Vitro Research Products
Please be aware that all articles and product information presented on BenchChem are intended solely for informational purposes. The products available for purchase on BenchChem are specifically designed for in-vitro studies, which are conducted outside of living organisms. In-vitro studies, derived from the Latin term "in glass," involve experiments performed in controlled laboratory settings using cells or tissues. It is important to note that these products are not categorized as medicines or drugs, and they have not received approval from the FDA for the prevention, treatment, or cure of any medical condition, ailment, or disease. We must emphasize that any form of bodily introduction of these products into humans or animals is strictly prohibited by law. It is essential to adhere to these guidelines to ensure compliance with legal and ethical standards in research and experimentation.