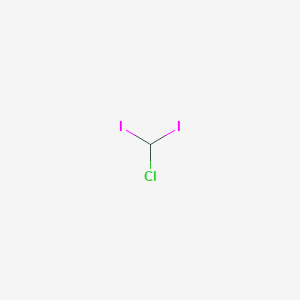
Chlorodiiodomethane
Overview
Description
Chlorodiiodomethane, also known as chloro(diiodo)methane, is a trihalomethane with the chemical formula CHClI₂. It is a colorless liquid that is primarily used in organic synthesis. This compound is of interest due to its unique reactivity and its role as a precursor in various chemical reactions.
Mechanism of Action
Target of Action
Chlorodiiodomethane, also known as Chloromethyl iodide, is primarily used in organic synthesis . It is a halomethane with the formula CH2ClI . The primary targets of this compound are organic compounds that undergo reactions such as cyclopropanation, Mannich reaction, aminomethylation, epoxidation, ring opening, and addition to terminal alkenes .
Mode of Action
This compound interacts with its targets through various chemical reactions. For instance, in the Simmon-Smith reaction (a type of cyclopropanation), this compound often replaces diiodomethane due to its higher yields and selectivity . It also reacts with organolithium compounds to give chloromethyl lithium (ClCH2Li) .
Biochemical Pathways
It is known to be involved in various organic synthesis reactions, including cyclopropanation, mannich reaction, aminomethylation, epoxidation, ring opening, and addition to terminal alkenes . These reactions are fundamental to various biochemical processes, potentially affecting multiple pathways.
Result of Action
The molecular and cellular effects of this compound’s action would depend on the specific reactions it’s involved in. For instance, in the Simmon-Smith reaction, it can facilitate the formation of cyclopropane rings . In reactions with organolithium compounds, it can lead to the production of chloromethyl lithium .
Biochemical Analysis
Biochemical Properties
Chlorodiiodomethane has a molecular weight of 302.28 . It is sparingly soluble in chloroform and slightly soluble in methanol
Cellular Effects
This compound has been found to induce DNA damage in Chinese hamster ovary (CHO) cells
Molecular Mechanism
Preparation Methods
Synthetic Routes and Reaction Conditions: Chlorodiiodomethane can be synthesized through the halogenation of methylene iodide (diiodomethane) with chlorine. The reaction typically involves the controlled addition of chlorine gas to diiodomethane in the presence of a suitable solvent, such as carbon tetrachloride, under controlled temperature conditions to avoid over-chlorination.
Industrial Production Methods: Industrial production of this compound follows similar principles but on a larger scale. The process involves the use of industrial reactors where chlorine gas is introduced to diiodomethane under controlled conditions to ensure high yield and purity of the product.
Types of Reactions:
Photolysis: this compound undergoes photolysis, where it is broken down by ultraviolet light to form reactive intermediates.
Substitution Reactions: It can participate in nucleophilic substitution reactions, where the chlorine or iodine atoms are replaced by other nucleophiles.
Addition Reactions: this compound can add to alkenes and alkynes, forming various addition products.
Common Reagents and Conditions:
Photolysis: Ultraviolet light is used to initiate the breakdown of this compound.
Nucleophilic Substitution: Common nucleophiles include hydroxide ions, alkoxide ions, and amines.
Addition Reactions: Catalysts such as palladium or platinum may be used to facilitate the addition reactions.
Major Products:
Photolysis: Produces reactive intermediates such as chloroiodomethyl radicals.
Substitution Reactions: Produces substituted methanes, depending on the nucleophile used.
Addition Reactions: Produces various addition products, including halogenated alkanes and alkenes.
Scientific Research Applications
Chlorodiiodomethane has several applications in scientific research:
Organic Synthesis: It is used as a reagent in the synthesis of complex organic molecules.
Photochemistry: Its photolysis properties make it useful in studying photochemical reactions and mechanisms.
Material Science: It is used in the preparation of halogenated polymers and other materials.
Environmental Chemistry: It is studied for its role in atmospheric chemistry and its potential environmental impact.
Comparison with Similar Compounds
Chloroiodomethane (CH₂ClI): Similar in structure but with one iodine atom replaced by a hydrogen atom.
Diiodomethane (CH₂I₂): Contains two iodine atoms and no chlorine atom.
Bromoiodomethane (CH₂BrI): Contains one bromine atom and one iodine atom.
Uniqueness: Chlorodiiodomethane is unique due to its combination of chlorine and iodine atoms, which gives it distinct reactivity compared to other halomethanes. Its ability to undergo photolysis and form reactive intermediates makes it particularly useful in photochemical studies and organic synthesis.
Properties
IUPAC Name |
chloro(diiodo)methane | |
---|---|---|
Source | PubChem | |
URL | https://pubchem.ncbi.nlm.nih.gov | |
Description | Data deposited in or computed by PubChem | |
InChI |
InChI=1S/CHClI2/c2-1(3)4/h1H | |
Source | PubChem | |
URL | https://pubchem.ncbi.nlm.nih.gov | |
Description | Data deposited in or computed by PubChem | |
InChI Key |
RYPFDEIVUZVDCE-UHFFFAOYSA-N | |
Source | PubChem | |
URL | https://pubchem.ncbi.nlm.nih.gov | |
Description | Data deposited in or computed by PubChem | |
Canonical SMILES |
C(Cl)(I)I | |
Source | PubChem | |
URL | https://pubchem.ncbi.nlm.nih.gov | |
Description | Data deposited in or computed by PubChem | |
Molecular Formula |
CHClI2 | |
Source | PubChem | |
URL | https://pubchem.ncbi.nlm.nih.gov | |
Description | Data deposited in or computed by PubChem | |
DSSTOX Substance ID |
DTXSID20213251 | |
Record name | Chlorodiiodomethane | |
Source | EPA DSSTox | |
URL | https://comptox.epa.gov/dashboard/DTXSID20213251 | |
Description | DSSTox provides a high quality public chemistry resource for supporting improved predictive toxicology. | |
Molecular Weight |
302.28 g/mol | |
Source | PubChem | |
URL | https://pubchem.ncbi.nlm.nih.gov | |
Description | Data deposited in or computed by PubChem | |
CAS No. |
638-73-3 | |
Record name | Chlorodiiodomethane | |
Source | ChemIDplus | |
URL | https://pubchem.ncbi.nlm.nih.gov/substance/?source=chemidplus&sourceid=0000638733 | |
Description | ChemIDplus is a free, web search system that provides access to the structure and nomenclature authority files used for the identification of chemical substances cited in National Library of Medicine (NLM) databases, including the TOXNET system. | |
Record name | Chlorodiiodomethane | |
Source | EPA DSSTox | |
URL | https://comptox.epa.gov/dashboard/DTXSID20213251 | |
Description | DSSTox provides a high quality public chemistry resource for supporting improved predictive toxicology. | |
Q1: What are the main health concerns surrounding chlorodiiodomethane found in drinking water?
A1: this compound (CDIM) is an iodinated disinfection byproduct (I-DBP) found in drinking water treated with chlorine or chloramine. While often present at lower concentrations than other DBPs, CDIM exhibits higher cytotoxicity and genotoxicity compared to its brominated and chlorinated counterparts [, ]. Genotoxicity refers to its ability to damage DNA, potentially leading to mutations and increasing cancer risk.
Q2: Can cooking with iodized salt and chloraminated water contribute to this compound exposure?
A3: Yes, recent research reveals an overlooked source of CDIM exposure: cooking with iodized table salt and chloraminated tap water []. During cooking, the iodide in the salt can react with chloramine residuals and organic matter in food, generating I-DBPs, including CDIM. Notably, this formation is significantly reduced when iodized salt is added after cooking.
Q3: How does this compound's structure influence its toxicity compared to other trihalomethanes?
A4: The presence of iodine in trihalomethanes generally increases their cytotoxicity and genotoxicity compared to their brominated or chlorinated counterparts []. For example, iodoacetic acid is considerably more toxic than its brominated analogue, bromoacetic acid. This difference likely stems from the weaker carbon-iodine bond, making it easier to break and potentially leading to more reactive species within cells.
Q4: Are there reliable analytical methods to detect and quantify this compound in complex matrices like food?
A5: Accurately measuring CDIM in complex matrices like cooked food can be challenging. A recent study developed a sensitive and reproducible method using gas chromatography-mass spectrometry/mass spectrometry (GC-MS/MS) after sample cleanup and extraction []. This method successfully quantified CDIM and other I-THMs in pasta cooked with iodized salt and chloraminated water.
Q5: What is the environmental fate of this compound?
A5: While the provided research focuses on CDIM formation and toxicity, it lacks information on its environmental fate and degradation pathways. Further research is crucial to understand its persistence in the environment, potential for bioaccumulation, and any ecological risks associated with its presence in water sources.
Q6: What are the implications of this compound's genotoxicity for human health?
A7: CDIM's demonstrated genotoxicity in mammalian cells raises concerns about its potential long-term health effects [, ]. Genotoxic substances can damage DNA, increasing the risk of mutations that may lead to cancer development. Further research, including epidemiological studies, is necessary to determine the actual human health risks associated with chronic, low-level exposure to CDIM in drinking water.
Disclaimer and Information on In-Vitro Research Products
Please be aware that all articles and product information presented on BenchChem are intended solely for informational purposes. The products available for purchase on BenchChem are specifically designed for in-vitro studies, which are conducted outside of living organisms. In-vitro studies, derived from the Latin term "in glass," involve experiments performed in controlled laboratory settings using cells or tissues. It is important to note that these products are not categorized as medicines or drugs, and they have not received approval from the FDA for the prevention, treatment, or cure of any medical condition, ailment, or disease. We must emphasize that any form of bodily introduction of these products into humans or animals is strictly prohibited by law. It is essential to adhere to these guidelines to ensure compliance with legal and ethical standards in research and experimentation.