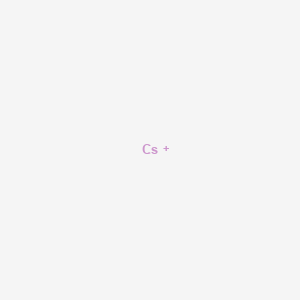
Cesium, ion (Cs1+)
Overview
Description
Cesium, ion (Cs1+) (Cs⁺) is a monovalent cation derived from the element cesium, which is an alkali metal with the atomic number 55. Cesium is known for its high reactivity and is one of the most electropositive and alkaline elements. Cesium, ion (Cs1+)s are commonly found in various chemical compounds and have significant applications in different fields due to their unique properties.
Preparation Methods
Synthetic Routes and Reaction Conditions
Cesium, ion (Cs1+)s can be prepared through several synthetic routes. One common method involves the dissolution of cesium salts, such as cesium chloride (CsCl) or cesium sulfate (Cs₂SO₄), in water. The dissolution process releases cesium ions into the solution. Another method involves the ion exchange process, where cesium ions are exchanged with other cations in a solution using ion exchange resins.
Industrial Production Methods
Industrially, cesium ions are often produced from cesium-bearing minerals such as pollucite (Cs₂Al₂Si₄O₁₂·2H₂O). The mineral is first crushed and then subjected to a series of chemical treatments, including acid leaching and precipitation, to extract cesium ions. The extracted cesium ions are then purified and converted into various cesium compounds for industrial use .
Chemical Reactions Analysis
Types of Reactions
Cesium, ion (Cs1+)s undergo various types of chemical reactions, including:
Oxidation-Reduction Reactions: this compounds can participate in redox reactions, where they can be reduced to elemental cesium or oxidized to higher oxidation states.
Substitution Reactions: this compounds can replace other cations in compounds through substitution reactions.
Complexation Reactions: this compounds can form complexes with various ligands, enhancing their stability and solubility.
Common Reagents and Conditions
Common reagents used in reactions involving cesium ions include cesium carbonate (Cs₂CO₃), cesium hydroxide (CsOH), and cesium chloride (CsCl). These reactions typically occur under aqueous conditions, and the choice of reagent and conditions depends on the desired reaction and product.
Major Products Formed
Major products formed from reactions involving cesium ions include cesium salts (e.g., cesium chloride, cesium sulfate), cesium hydroxide, and various cesium complexes. These products have diverse applications in different fields .
Scientific Research Applications
Cesium, ion (Cs1+)s have a
Properties
IUPAC Name |
cesium(1+) | |
---|---|---|
Source | PubChem | |
URL | https://pubchem.ncbi.nlm.nih.gov | |
Description | Data deposited in or computed by PubChem | |
InChI |
InChI=1S/Cs/q+1 | |
Source | PubChem | |
URL | https://pubchem.ncbi.nlm.nih.gov | |
Description | Data deposited in or computed by PubChem | |
InChI Key |
NCMHKCKGHRPLCM-UHFFFAOYSA-N | |
Source | PubChem | |
URL | https://pubchem.ncbi.nlm.nih.gov | |
Description | Data deposited in or computed by PubChem | |
Canonical SMILES |
[Cs+] | |
Source | PubChem | |
URL | https://pubchem.ncbi.nlm.nih.gov | |
Description | Data deposited in or computed by PubChem | |
Molecular Formula |
Cs+ | |
Source | PubChem | |
URL | https://pubchem.ncbi.nlm.nih.gov | |
Description | Data deposited in or computed by PubChem | |
DSSTOX Substance ID |
DTXSID601318357 | |
Record name | Cesium(1+) | |
Source | EPA DSSTox | |
URL | https://comptox.epa.gov/dashboard/DTXSID601318357 | |
Description | DSSTox provides a high quality public chemistry resource for supporting improved predictive toxicology. | |
Molecular Weight |
132.9054520 g/mol | |
Source | PubChem | |
URL | https://pubchem.ncbi.nlm.nih.gov | |
Description | Data deposited in or computed by PubChem | |
CAS No. |
18459-37-5 | |
Record name | Cesium(1+) | |
Source | CAS Common Chemistry | |
URL | https://commonchemistry.cas.org/detail?cas_rn=18459-37-5 | |
Description | CAS Common Chemistry is an open community resource for accessing chemical information. Nearly 500,000 chemical substances from CAS REGISTRY cover areas of community interest, including common and frequently regulated chemicals, and those relevant to high school and undergraduate chemistry classes. This chemical information, curated by our expert scientists, is provided in alignment with our mission as a division of the American Chemical Society. | |
Explanation | The data from CAS Common Chemistry is provided under a CC-BY-NC 4.0 license, unless otherwise stated. | |
Record name | Cesium cation | |
Source | ChemIDplus | |
URL | https://pubchem.ncbi.nlm.nih.gov/substance/?source=chemidplus&sourceid=0018459375 | |
Description | ChemIDplus is a free, web search system that provides access to the structure and nomenclature authority files used for the identification of chemical substances cited in National Library of Medicine (NLM) databases, including the TOXNET system. | |
Record name | Cesium(1+) | |
Source | EPA DSSTox | |
URL | https://comptox.epa.gov/dashboard/DTXSID601318357 | |
Description | DSSTox provides a high quality public chemistry resource for supporting improved predictive toxicology. | |
Record name | CESIUM CATION | |
Source | FDA Global Substance Registration System (GSRS) | |
URL | https://gsrs.ncats.nih.gov/ginas/app/beta/substances/LCC4BG14DM | |
Description | The FDA Global Substance Registration System (GSRS) enables the efficient and accurate exchange of information on what substances are in regulated products. Instead of relying on names, which vary across regulatory domains, countries, and regions, the GSRS knowledge base makes it possible for substances to be defined by standardized, scientific descriptions. | |
Explanation | Unless otherwise noted, the contents of the FDA website (www.fda.gov), both text and graphics, are not copyrighted. They are in the public domain and may be republished, reprinted and otherwise used freely by anyone without the need to obtain permission from FDA. Credit to the U.S. Food and Drug Administration as the source is appreciated but not required. | |
Synthesis routes and methods
Procedure details
Disclaimer and Information on In-Vitro Research Products
Please be aware that all articles and product information presented on BenchChem are intended solely for informational purposes. The products available for purchase on BenchChem are specifically designed for in-vitro studies, which are conducted outside of living organisms. In-vitro studies, derived from the Latin term "in glass," involve experiments performed in controlled laboratory settings using cells or tissues. It is important to note that these products are not categorized as medicines or drugs, and they have not received approval from the FDA for the prevention, treatment, or cure of any medical condition, ailment, or disease. We must emphasize that any form of bodily introduction of these products into humans or animals is strictly prohibited by law. It is essential to adhere to these guidelines to ensure compliance with legal and ethical standards in research and experimentation.