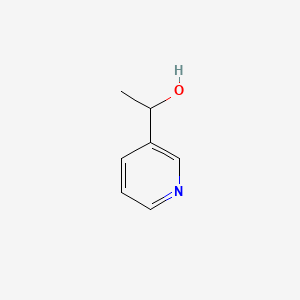
3-(1-Hydroxyethyl)pyridine
Overview
Description
3-(1-Hydroxyethyl)pyridine is an organic compound with the molecular formula C7H9NO. It is a derivative of pyridine, featuring a hydroxyethyl group attached to the third carbon of the pyridine ring. This compound is known for its applications in various fields, including organic synthesis and medicinal chemistry.
Preparation Methods
Synthetic Routes and Reaction Conditions: The synthesis of 3-(1-Hydroxyethyl)pyridine typically involves the reaction of pyridine with ethylene oxide under acidic or basic conditions. Another method includes the reduction of 3-acetylpyridine using sodium borohydride or lithium aluminum hydride to yield this compound .
Industrial Production Methods: Industrial production of this compound often employs catalytic hydrogenation of 3-acetylpyridine. This method is preferred due to its efficiency and scalability .
Types of Reactions:
Oxidation: this compound can undergo oxidation to form 3-acetylpyridine.
Reduction: The compound can be reduced to 3-ethylpyridine using strong reducing agents like lithium aluminum hydride.
Common Reagents and Conditions:
Oxidation: Potassium permanganate in an acidic medium.
Reduction: Lithium aluminum hydride in anhydrous ether.
Substitution: Nucleophiles such as halides or amines in the presence of a base.
Major Products:
Oxidation: 3-Acetylpyridine.
Reduction: 3-Ethylpyridine.
Substitution: Various substituted pyridines depending on the nucleophile used.
Scientific Research Applications
3-(1-Hydroxyethyl)pyridine has several applications in scientific research:
Mechanism of Action
The mechanism of action of 3-(1-Hydroxyethyl)pyridine involves its interaction with various molecular targets. It can act as a ligand, binding to specific receptors or enzymes and modulating their activity. The hydroxyethyl group allows for hydrogen bonding, which can enhance its binding affinity to biological targets .
Comparison with Similar Compounds
3-Acetylpyridine: Similar structure but with a ketone group instead of a hydroxyethyl group.
3-Ethylpyridine: Similar structure but with an ethyl group instead of a hydroxyethyl group.
3-Hydroxypyridine: Similar structure but with a hydroxyl group directly attached to the pyridine ring.
Uniqueness: 3-(1-Hydroxyethyl)pyridine is unique due to the presence of the hydroxyethyl group, which imparts distinct chemical and biological properties. This group allows for specific interactions in chemical reactions and biological systems, making it a valuable compound in various applications .
Properties
IUPAC Name |
1-pyridin-3-ylethanol | |
---|---|---|
Source | PubChem | |
URL | https://pubchem.ncbi.nlm.nih.gov | |
Description | Data deposited in or computed by PubChem | |
InChI |
InChI=1S/C7H9NO/c1-6(9)7-3-2-4-8-5-7/h2-6,9H,1H3 | |
Source | PubChem | |
URL | https://pubchem.ncbi.nlm.nih.gov | |
Description | Data deposited in or computed by PubChem | |
InChI Key |
QMDUEBURHKSKDG-UHFFFAOYSA-N | |
Source | PubChem | |
URL | https://pubchem.ncbi.nlm.nih.gov | |
Description | Data deposited in or computed by PubChem | |
Canonical SMILES |
CC(C1=CN=CC=C1)O | |
Source | PubChem | |
URL | https://pubchem.ncbi.nlm.nih.gov | |
Description | Data deposited in or computed by PubChem | |
Molecular Formula |
C7H9NO | |
Source | PubChem | |
URL | https://pubchem.ncbi.nlm.nih.gov | |
Description | Data deposited in or computed by PubChem | |
DSSTOX Substance ID |
DTXSID20909829 | |
Record name | 1-(Pyridin-3-yl)ethan-1-ol | |
Source | EPA DSSTox | |
URL | https://comptox.epa.gov/dashboard/DTXSID20909829 | |
Description | DSSTox provides a high quality public chemistry resource for supporting improved predictive toxicology. | |
Molecular Weight |
123.15 g/mol | |
Source | PubChem | |
URL | https://pubchem.ncbi.nlm.nih.gov | |
Description | Data deposited in or computed by PubChem | |
CAS No. |
4754-27-2, 10593-35-8 | |
Record name | α-Methyl-3-pyridinemethanol | |
Source | CAS Common Chemistry | |
URL | https://commonchemistry.cas.org/detail?cas_rn=4754-27-2 | |
Description | CAS Common Chemistry is an open community resource for accessing chemical information. Nearly 500,000 chemical substances from CAS REGISTRY cover areas of community interest, including common and frequently regulated chemicals, and those relevant to high school and undergraduate chemistry classes. This chemical information, curated by our expert scientists, is provided in alignment with our mission as a division of the American Chemical Society. | |
Explanation | The data from CAS Common Chemistry is provided under a CC-BY-NC 4.0 license, unless otherwise stated. | |
Record name | 1-(3-Pyridyl)ethanol | |
Source | ChemIDplus | |
URL | https://pubchem.ncbi.nlm.nih.gov/substance/?source=chemidplus&sourceid=0004754272 | |
Description | ChemIDplus is a free, web search system that provides access to the structure and nomenclature authority files used for the identification of chemical substances cited in National Library of Medicine (NLM) databases, including the TOXNET system. | |
Record name | 3-Pyridinemethanol, alpha-methyl-, (+-)- | |
Source | ChemIDplus | |
URL | https://pubchem.ncbi.nlm.nih.gov/substance/?source=chemidplus&sourceid=0010593358 | |
Description | ChemIDplus is a free, web search system that provides access to the structure and nomenclature authority files used for the identification of chemical substances cited in National Library of Medicine (NLM) databases, including the TOXNET system. | |
Record name | 4754-27-2 | |
Source | DTP/NCI | |
URL | https://dtp.cancer.gov/dtpstandard/servlet/dwindex?searchtype=NSC&outputformat=html&searchlist=526489 | |
Description | The NCI Development Therapeutics Program (DTP) provides services and resources to the academic and private-sector research communities worldwide to facilitate the discovery and development of new cancer therapeutic agents. | |
Explanation | Unless otherwise indicated, all text within NCI products is free of copyright and may be reused without our permission. Credit the National Cancer Institute as the source. | |
Record name | 1-(Pyridin-3-yl)ethan-1-ol | |
Source | EPA DSSTox | |
URL | https://comptox.epa.gov/dashboard/DTXSID20909829 | |
Description | DSSTox provides a high quality public chemistry resource for supporting improved predictive toxicology. | |
Record name | α-methylpyridine-3-methanol | |
Source | European Chemicals Agency (ECHA) | |
URL | https://echa.europa.eu/substance-information/-/substanceinfo/100.022.982 | |
Description | The European Chemicals Agency (ECHA) is an agency of the European Union which is the driving force among regulatory authorities in implementing the EU's groundbreaking chemicals legislation for the benefit of human health and the environment as well as for innovation and competitiveness. | |
Explanation | Use of the information, documents and data from the ECHA website is subject to the terms and conditions of this Legal Notice, and subject to other binding limitations provided for under applicable law, the information, documents and data made available on the ECHA website may be reproduced, distributed and/or used, totally or in part, for non-commercial purposes provided that ECHA is acknowledged as the source: "Source: European Chemicals Agency, http://echa.europa.eu/". Such acknowledgement must be included in each copy of the material. ECHA permits and encourages organisations and individuals to create links to the ECHA website under the following cumulative conditions: Links can only be made to webpages that provide a link to the Legal Notice page. | |
Record name | 1-(3-PYRIDYL)ETHANOL | |
Source | FDA Global Substance Registration System (GSRS) | |
URL | https://gsrs.ncats.nih.gov/ginas/app/beta/substances/H6E9JKE977 | |
Description | The FDA Global Substance Registration System (GSRS) enables the efficient and accurate exchange of information on what substances are in regulated products. Instead of relying on names, which vary across regulatory domains, countries, and regions, the GSRS knowledge base makes it possible for substances to be defined by standardized, scientific descriptions. | |
Explanation | Unless otherwise noted, the contents of the FDA website (www.fda.gov), both text and graphics, are not copyrighted. They are in the public domain and may be republished, reprinted and otherwise used freely by anyone without the need to obtain permission from FDA. Credit to the U.S. Food and Drug Administration as the source is appreciated but not required. | |
Synthesis routes and methods I
Procedure details
Synthesis routes and methods II
Procedure details
Synthesis routes and methods III
Procedure details
Synthesis routes and methods IV
Procedure details
Synthesis routes and methods V
Procedure details
Retrosynthesis Analysis
AI-Powered Synthesis Planning: Our tool employs the Template_relevance Pistachio, Template_relevance Bkms_metabolic, Template_relevance Pistachio_ringbreaker, Template_relevance Reaxys, Template_relevance Reaxys_biocatalysis model, leveraging a vast database of chemical reactions to predict feasible synthetic routes.
One-Step Synthesis Focus: Specifically designed for one-step synthesis, it provides concise and direct routes for your target compounds, streamlining the synthesis process.
Accurate Predictions: Utilizing the extensive PISTACHIO, BKMS_METABOLIC, PISTACHIO_RINGBREAKER, REAXYS, REAXYS_BIOCATALYSIS database, our tool offers high-accuracy predictions, reflecting the latest in chemical research and data.
Strategy Settings
Precursor scoring | Relevance Heuristic |
---|---|
Min. plausibility | 0.01 |
Model | Template_relevance |
Template Set | Pistachio/Bkms_metabolic/Pistachio_ringbreaker/Reaxys/Reaxys_biocatalysis |
Top-N result to add to graph | 6 |
Feasible Synthetic Routes
Q1: What is the significance of 3-(1-Hydroxyethyl)pyridine in the context of natural product isolation?
A1: The research paper "New Iridoid Glucoside from Pterocephalus hookeri" [] describes the isolation of a novel iridoid glucoside from the Pterocephalus hookeri plant. This new compound is identified as a 5-[this compound],7-loganin ester. This discovery marks the first reported instance of this compound existing as a structural component within a naturally occurring iridoid glucoside. This finding broadens our understanding of the chemical diversity present in Pterocephalus hookeri and highlights the potential of this plant as a source of novel bioactive compounds.
Q2: Has the interaction of this compound with other molecules been investigated computationally?
A2: While the provided research doesn't directly explore the biological activity of this compound, a separate study titled "DFT Study of 6-amino-3-(1-hydroxyethyl) pyridine-2,4-diol (AHP) Adsorption on Coronene" [] investigates the adsorption behavior of a related compound, 6-amino-3-(1-hydroxyethyl) pyridine-2,4-diol (AHP), on coronene using Density Functional Theory (DFT) calculations. This study provides insights into the potential interactions of substituted this compound derivatives with aromatic surfaces, which could be relevant for understanding their binding affinities to biological targets.
Disclaimer and Information on In-Vitro Research Products
Please be aware that all articles and product information presented on BenchChem are intended solely for informational purposes. The products available for purchase on BenchChem are specifically designed for in-vitro studies, which are conducted outside of living organisms. In-vitro studies, derived from the Latin term "in glass," involve experiments performed in controlled laboratory settings using cells or tissues. It is important to note that these products are not categorized as medicines or drugs, and they have not received approval from the FDA for the prevention, treatment, or cure of any medical condition, ailment, or disease. We must emphasize that any form of bodily introduction of these products into humans or animals is strictly prohibited by law. It is essential to adhere to these guidelines to ensure compliance with legal and ethical standards in research and experimentation.