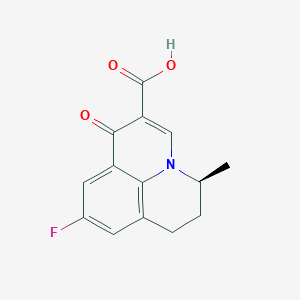
(S)-Flumequine
Overview
Description
2’-O-Methylguanosine: is a modified nucleoside that is produced in transfer ribonucleic acids by the action of transfer ribonucleic acid guanosine-2’-O-methyltransferase. It is characterized by the substitution of a hydrogen atom on the hydroxyl group at the C-2’ position with a methyl group . This modification plays a crucial role in the stability and function of ribonucleic acids, particularly in the context of transfer ribonucleic acids and messenger ribonucleic acids.
Preparation Methods
Synthetic Routes and Reaction Conditions: The synthesis of 2’-O-Methylguanosine typically involves the methylation of guanosine at the 2’-hydroxyl group. One common method is the use of methyl iodide or methyl triflate as the methylating agent in the presence of a base such as sodium hydride or potassium carbonate . The reaction is usually carried out in an aprotic solvent like dimethylformamide or dimethyl sulfoxide at elevated temperatures.
Industrial Production Methods: In an industrial setting, the production of 2’-O-Methylguanosine can be scaled up using similar methylation reactions. The process involves the purification of the product through crystallization or chromatography to achieve high purity levels required for research and pharmaceutical applications .
Chemical Reactions Analysis
Types of Reactions: 2’-O-Methylguanosine can undergo various chemical reactions, including:
Oxidation: The compound can be oxidized to form 2’-O-Methylguanine.
Reduction: Reduction reactions can convert it back to guanosine.
Substitution: The methyl group at the 2’-position can be substituted with other functional groups under specific conditions.
Common Reagents and Conditions:
Oxidation: Common oxidizing agents include potassium permanganate and hydrogen peroxide.
Reduction: Reducing agents such as sodium borohydride or lithium aluminum hydride are used.
Substitution: Nucleophilic substitution reactions can be carried out using reagents like sodium azide or thiols.
Major Products:
Oxidation: 2’-O-Methylguanine.
Reduction: Guanosine.
Substitution: Various substituted guanosine derivatives depending on the nucleophile used.
Scientific Research Applications
2’-O-Methylguanosine has a wide range of applications in scientific research:
Mechanism of Action
The mechanism of action of 2’-O-Methylguanosine involves its incorporation into ribonucleic acids, where it influences the stability and function of the ribonucleic acid molecules. The methylation at the 2’-position enhances the resistance of ribonucleic acids to enzymatic degradation and improves their binding affinity to proteins involved in ribonucleic acid processing . This modification can also induce apoptotic changes in cells by affecting the function of transfer ribonucleic acids and messenger ribonucleic acids .
Comparison with Similar Compounds
7-Methylguanosine: Another modified nucleoside with a methyl group at the 7-position of guanine.
2’-O-Methyladenosine: Similar to 2’-O-Methylguanosine but with a methyl group at the 2’-position of adenosine.
2’-O-Methylcytidine: A methylated cytidine at the 2’-position.
Uniqueness: 2’-O-Methylguanosine is unique due to its specific role in stabilizing ribonucleic acids and its ability to modulate ribonucleic acid function. Its methylation at the 2’-position provides distinct advantages in terms of ribonucleic acid stability and resistance to degradation compared to other methylated nucleosides .
Properties
IUPAC Name |
(12S)-7-fluoro-12-methyl-4-oxo-1-azatricyclo[7.3.1.05,13]trideca-2,5,7,9(13)-tetraene-3-carboxylic acid | |
---|---|---|
Source | PubChem | |
URL | https://pubchem.ncbi.nlm.nih.gov | |
Description | Data deposited in or computed by PubChem | |
InChI |
InChI=1S/C14H12FNO3/c1-7-2-3-8-4-9(15)5-10-12(8)16(7)6-11(13(10)17)14(18)19/h4-7H,2-3H2,1H3,(H,18,19)/t7-/m0/s1 | |
Source | PubChem | |
URL | https://pubchem.ncbi.nlm.nih.gov | |
Description | Data deposited in or computed by PubChem | |
InChI Key |
DPSPPJIUMHPXMA-ZETCQYMHSA-N | |
Source | PubChem | |
URL | https://pubchem.ncbi.nlm.nih.gov | |
Description | Data deposited in or computed by PubChem | |
Canonical SMILES |
CC1CCC2=C3N1C=C(C(=O)C3=CC(=C2)F)C(=O)O | |
Source | PubChem | |
URL | https://pubchem.ncbi.nlm.nih.gov | |
Description | Data deposited in or computed by PubChem | |
Isomeric SMILES |
C[C@H]1CCC2=C3N1C=C(C(=O)C3=CC(=C2)F)C(=O)O | |
Source | PubChem | |
URL | https://pubchem.ncbi.nlm.nih.gov | |
Description | Data deposited in or computed by PubChem | |
Molecular Formula |
C14H12FNO3 | |
Source | PubChem | |
URL | https://pubchem.ncbi.nlm.nih.gov | |
Description | Data deposited in or computed by PubChem | |
Molecular Weight |
261.25 g/mol | |
Source | PubChem | |
URL | https://pubchem.ncbi.nlm.nih.gov | |
Description | Data deposited in or computed by PubChem | |
CAS No. |
202349-45-9 | |
Record name | Flumequine, (S)- | |
Source | ChemIDplus | |
URL | https://pubchem.ncbi.nlm.nih.gov/substance/?source=chemidplus&sourceid=0202349459 | |
Description | ChemIDplus is a free, web search system that provides access to the structure and nomenclature authority files used for the identification of chemical substances cited in National Library of Medicine (NLM) databases, including the TOXNET system. | |
Record name | FLUMEQUINE, (S)- | |
Source | FDA Global Substance Registration System (GSRS) | |
URL | https://gsrs.ncats.nih.gov/ginas/app/beta/substances/BRD84T215S | |
Description | The FDA Global Substance Registration System (GSRS) enables the efficient and accurate exchange of information on what substances are in regulated products. Instead of relying on names, which vary across regulatory domains, countries, and regions, the GSRS knowledge base makes it possible for substances to be defined by standardized, scientific descriptions. | |
Explanation | Unless otherwise noted, the contents of the FDA website (www.fda.gov), both text and graphics, are not copyrighted. They are in the public domain and may be republished, reprinted and otherwise used freely by anyone without the need to obtain permission from FDA. Credit to the U.S. Food and Drug Administration as the source is appreciated but not required. | |
Retrosynthesis Analysis
AI-Powered Synthesis Planning: Our tool employs the Template_relevance Pistachio, Template_relevance Bkms_metabolic, Template_relevance Pistachio_ringbreaker, Template_relevance Reaxys, Template_relevance Reaxys_biocatalysis model, leveraging a vast database of chemical reactions to predict feasible synthetic routes.
One-Step Synthesis Focus: Specifically designed for one-step synthesis, it provides concise and direct routes for your target compounds, streamlining the synthesis process.
Accurate Predictions: Utilizing the extensive PISTACHIO, BKMS_METABOLIC, PISTACHIO_RINGBREAKER, REAXYS, REAXYS_BIOCATALYSIS database, our tool offers high-accuracy predictions, reflecting the latest in chemical research and data.
Strategy Settings
Precursor scoring | Relevance Heuristic |
---|---|
Min. plausibility | 0.01 |
Model | Template_relevance |
Template Set | Pistachio/Bkms_metabolic/Pistachio_ringbreaker/Reaxys/Reaxys_biocatalysis |
Top-N result to add to graph | 6 |
Feasible Synthetic Routes
Q1: What is the significance of using water as a solvent in the synthesis of (S)-Flumequine?
A1: Utilizing water as a solvent in the asymmetric hydrogenation of quinolines to produce this compound offers significant advantages in terms of environmental friendliness and cost-effectiveness [, ]. This approach aligns with green chemistry principles by reducing reliance on organic solvents, which can be toxic and environmentally harmful. Furthermore, water often simplifies reaction workup procedures and can enhance reaction rates in certain cases.
Q2: How does the structure of the chiral cationic Ru-diamine catalyst influence the enantioselectivity of this compound synthesis?
A2: The chiral environment provided by the η(6)-arene-N-tosylethylenediamine-Ru(II) complex plays a crucial role in achieving high enantioselectivity during the hydrogenation of quinolines to this compound []. DFT calculations suggest that a key interaction involves CH/π attraction between the η(6)-arene ligand of the catalyst and the fused phenyl ring of the dihydroquinoline intermediate. This interaction, along with the influence of the TfO(-) anion, favors the formation of the (S)-enantiomer by stabilizing the transition state leading to its production.
Q3: Can you explain the "ionic and cascade reaction pathway" involved in the formation of this compound?
A3: The synthesis of this compound through this method doesn't occur in one step. Instead, it follows a multi-step process termed an "ionic and cascade reaction pathway" []. This involves:
Disclaimer and Information on In-Vitro Research Products
Please be aware that all articles and product information presented on BenchChem are intended solely for informational purposes. The products available for purchase on BenchChem are specifically designed for in-vitro studies, which are conducted outside of living organisms. In-vitro studies, derived from the Latin term "in glass," involve experiments performed in controlled laboratory settings using cells or tissues. It is important to note that these products are not categorized as medicines or drugs, and they have not received approval from the FDA for the prevention, treatment, or cure of any medical condition, ailment, or disease. We must emphasize that any form of bodily introduction of these products into humans or animals is strictly prohibited by law. It is essential to adhere to these guidelines to ensure compliance with legal and ethical standards in research and experimentation.