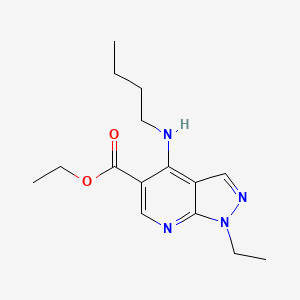
Cartazolate
Overview
Description
Cartazolate is a pyrazolopyridine derivative recognized for its pharmacological activity as a non-xanthine adenosine receptor antagonist and anxiolytic agent. Structurally, it belongs to the pyrazolo[3,4-b]pyridine family, a heterocyclic scaffold prevalent in over 60% of FDA-approved drugs due to its versatility in drug design . This compound primarily inhibits adenosine A2 receptors, with a Ki value of 2.0 μM, and demonstrates additional activity as a phosphodiesterase (PDE) inhibitor, contributing to its central nervous system (CNS) effects .
Preparation Methods
Traditional Condensation and Cyclization Methods
The foundational approach to synthesizing pyrazolo[3,4-b]pyridines, including Cartazolate, involves the condensation of N(1)-substituted 5-aminopyrazoles with 1,3-bis-electrophilic synthons. The Bare synthesis, a classical method, exemplifies this strategy .
Bare’s Two-Step Synthesis
In the Bare synthesis, 5-aminopyrazole (7 ) undergoes condensation with a masked 1,3-carbonyl compound (8 ), such as a β-ketoester or β-diketone, to form an intermediate (9 ). Subsequent cyclization under acidic conditions yields the pyrazolo[3,4-b]pyridine core (10 ) (Scheme 1) .
Key Reaction Conditions:
-
Step 1: Ethanol reflux, 12–24 hours.
-
Step 2: Concentrated HCl or H2SO4, 80–100°C, 2–4 hours.
-
Yield: ~50–60% (historically reported for analogous compounds) .
This method, while reliable, suffers from moderate yields and prolonged reaction times. However, it remains a benchmark for newer methodologies.
Base-Catalyzed Cyclization Approaches
Modern adaptations leverage base catalysis to enhance reaction efficiency. A representative protocol involves triethylamine (TEA)-mediated cyclization in ethanol .
Triethylamine-Promoted One-Pot Synthesis
A mixture of (arylhydrazono)methyl-4H-chromen-4-one (1a ), benzylamine (2a ), and malononitrile (3 ) in ethanol with 40% TEA at room temperature produces pyrazolopyridines in 65–80% yield within 8–12 hours .
Mechanistic Insights:
-
Michael addition of benzylamine to 1a forms intermediate A .
-
Malononitrile attack generates intermediate B , which undergoes cyclization via elimination and tautomerization .
Advantages:
-
Mild conditions (room temperature).
-
Avoids toxic solvents.
High-Pressure Synthesis Using Q-Tube Reactors
High-pressure methods using Q-tube reactors significantly accelerate reaction kinetics. A cyclocondensation protocol for pyrazolo[3,4-b]pyridines demonstrates this .
Q-Tube-Mediated Cyclocondensation
A mixture of 5-amino-2-phenyl-4H-pyrazol-3-one (1 ) and arylhydrazonals (2 ) in glacial acetic acid with ammonium acetate is heated at 160°C for 30 minutes under 180 psi pressure .
Parameter | Value |
---|---|
Temperature | 160°C |
Pressure | 180 psi |
Time | 30 minutes |
Yield | 85–92% |
Benefits:
Catalytic Methods Using Advanced Nanomaterials
Nanocatalysts like Alg@SBA-15/Fe3O4 have revolutionized pyrazolopyridine synthesis by enabling room-temperature reactions .
Alg@SBA-15/Fe3O4-Catalyzed Synthesis
The magnetic nanocatalyst (0.02 g) facilitates the reaction of aldehydes, amines, and malononitrile at room temperature, achieving 90–97% yield in 20–30 minutes .
Catalyst Characterization:
-
Surface Area: 650 m²/g (BET analysis).
-
Pore Size: 6.2 nm (mesoporous structure).
Reusability: Retains >90% activity after five cycles .
Recent Advances in Metal-Catalyzed Synthesis
Copper(II)-catalyzed methods represent cutting-edge innovations. A 2024 study reports a Cu(II)-mediated protocol for pyrazolo[3,4-b]pyridines .
Cu(II)-Catalyzed One-Pot Assembly
Using Cu(OAc)2·H2O (10 mol%) in DMF at 100°C, aryl aldehydes, hydrazines, and β-ketonitriles react to form pyrazolo[3,4-b]pyridines in 75–88% yield within 4 hours .
Substrate | Yield (%) |
---|---|
4-Chlorobenzaldehyde | 88 |
2-Nitrobenzaldehyde | 75 |
Advantages:
-
Broad substrate tolerance.
-
Ligand-free conditions.
Comparative Analysis of Preparation Methods
The following table evaluates key synthetic routes for this compound precursors:
Key Trends:
-
Efficiency: Nanocatalysts and Q-tube methods offer superior yields and shorter times.
-
Sustainability: Magnetic catalysts reduce waste via recyclability.
Chemical Reactions Analysis
Cartazolate undergoes various chemical reactions, including:
Oxidation: this compound can be oxidized under specific conditions to form corresponding oxidized derivatives.
Reduction: Reduction reactions can convert this compound into reduced forms, potentially altering its pharmacological properties.
Substitution: Substitution reactions can introduce different functional groups into the this compound molecule, modifying its chemical and biological activity.
Common reagents used in these reactions include oxidizing agents like potassium permanganate, reducing agents like sodium borohydride, and various nucleophiles for substitution reactions. The major products formed depend on the specific reaction conditions and reagents used .
Scientific Research Applications
Anxiolytic Effects
Several studies have demonstrated the efficacy of cartazolate in reducing anxiety symptoms:
- Sakalis et al. (1974) conducted an open-label study involving psychiatric inpatients where this compound (up to 200 mg/day) significantly reduced moderate-to-severe anxiety in 8 out of 10 patients, with no reported side effects .
- Sathananthan et al. (1976) reported similar findings in a study with patients suffering from severe psychotic depression. Out of 10 patients treated with this compound (up to 200 mg/day), four showed significant improvement .
These studies suggest that this compound offers non-sedative anxiolytic properties, distinguishing it from traditional benzodiazepines and barbiturates.
Antidepressant Properties
This compound has also been explored for its potential antidepressant effects:
- The compound was noted for its ability to alleviate symptoms of depression without sedative side effects, making it a valuable option for patients who may be sensitive to traditional antidepressants .
Comparative Potency
Research comparing this compound with other compounds indicates its relative potency:
- In studies examining the GABA A receptor binding affinity, this compound demonstrated an IC50 value of 500 nM , making it over four times more potent than tracazolate (IC50 of 2,200 nM) in inhibiting GABA A receptor binding . This suggests that lower doses of this compound may achieve similar therapeutic effects compared to tracazolate.
Summary of Clinical Studies
Study Reference | Patient Population | Dosage | Key Findings |
---|---|---|---|
Sakalis et al. (1974) | Psychiatric inpatients | Up to 200 mg/day | Significant reduction in anxiety; no side effects reported |
Sathananthan et al. (1976) | Patients with severe psychotic depression | Up to 200 mg/day | Four out of ten patients improved significantly |
Mechanism of Action
Cartazolate exerts its effects primarily through modulation of the gamma-aminobutyric acid A receptor at the barbiturate binding site. It enhances the receptor’s response to gamma-aminobutyric acid, leading to increased inhibitory neurotransmission. Additionally, this compound acts as an adenosine antagonist at the A1 and A2 subtypes, which may contribute to its anxiolytic effects. It also inhibits phosphodiesterase, leading to increased levels of cyclic adenosine monophosphate .
Comparison with Similar Compounds
Cartazolate shares structural and functional similarities with tracazolate and etazolate, two other pyrazolopyridine derivatives. Below is a detailed comparison based on receptor affinity, selectivity, and therapeutic applications:
Structural Similarities
All three compounds feature a pyrazolo[3,4-b]pyridine core, enabling interactions with adenosine receptors and PDE enzymes. Subtle substitutions (e.g., phenyl and methyl groups) modulate their receptor selectivity and potency .
Pharmacological Profiles
Receptor Affinity and Selectivity
Compound | A1 Receptor (Ki, μM) | A2 Receptor (Ki, μM) | PDE Inhibition |
---|---|---|---|
This compound | Not active at 50 μM | 2.0 | Potent |
Tracazolate | 0.5 (rat brain membranes) | 13 | Potent |
Etazolate | Not active at 50 μM | 3.9 | Potent |
- This compound : Exhibits the highest potency for A2 receptors but lacks A1 affinity in brain membrane assays .
- Etazolate : Intermediate A2 affinity but shares this compound’s inactivity at A1 receptors .
Mechanism of Anxiolytic Activity
All three compounds enhance benzodiazepine binding to GABAA receptors, implicating GABAergic modulation in their anxiolytic effects. However, their adenosine receptor antagonism and PDE inhibition likely synergize to amplify therapeutic outcomes .
Additional Pharmacological Activities
All three compounds inhibit PDE enzymes, increasing intracellular cAMP/cGMP levels. This property may augment their anxiolytic effects and broaden therapeutic utility in cardiovascular and inflammatory disorders .
Data Tables
Table 1: Key Pharmacological Parameters
Parameter | This compound | Tracazolate | Etazolate |
---|---|---|---|
A1 Ki (μM) | Inactive | 0.5 | Inactive |
A2 Ki (μM) | 2.0 | 13 | 3.9 |
PDE Inhibition | Yes | Yes | Yes |
Anxiolytic Activity | Yes | Yes | Yes |
Table 2: Therapeutic Indications
Biological Activity
Cartazolate, a compound belonging to the pyrazolopyridine class, has garnered attention for its biological activity, particularly its anxiolytic and antidepressant properties. This article provides a comprehensive overview of the biological mechanisms, research findings, and case studies associated with this compound.
Overview of this compound
This compound (chemical identifier: 34966-41-1) acts primarily as a positive allosteric modulator at the gamma-aminobutyric acid A (GABA) receptor, specifically at the barbiturate binding site. Additionally, it functions as an adenosine antagonist at A1 and A2 subtypes and a phosphodiesterase inhibitor , which contributes to its therapeutic potential in treating anxiety and depression .
The biological activity of this compound can be summarized through its interaction with key neurotransmitter systems:
- GABA Receptor Modulation : By enhancing GABA receptor activity, this compound increases inhibitory neurotransmission in the central nervous system (CNS), leading to anxiolytic effects. It has been shown to have a higher potency compared to similar compounds, such as Tracazolate, with IC values indicating that this compound is over four times more effective in inhibiting GABA receptor binding .
- Adenosine Receptor Antagonism : Its role as an adenosine antagonist may further contribute to its anxiolytic effects by modulating neuronal excitability and neurotransmitter release .
- Phosphodiesterase Inhibition : This action leads to increased levels of cyclic adenosine monophosphate (cAMP), which can enhance neuronal signaling pathways associated with mood regulation.
Research Findings
Several studies have investigated the efficacy and safety of this compound in clinical settings:
- Anxiety Treatment : In a small open-label study conducted by Sakalis et al. (1974), this compound was administered to psychiatric inpatients at doses up to 200 mg per day. The results indicated significant reductions in moderate-to-severe anxiety symptoms in 8 out of 10 patients, with no reported side effects .
- Depression Management : Another study by Sathananthan et al. (1976) involved 10 patients suffering from severe psychotic depression who were treated with this compound. Four patients showed significant improvement, while none of the six non-responders benefited from imipramine, a common tricyclic antidepressant .
- Comparative Potency Studies : Wong et al. (1984) demonstrated that this compound's binding affinity for the GABA receptor was significantly higher than that of Tracazolate, suggesting that lower doses may be required for similar therapeutic effects .
Case Studies
The following case studies highlight the clinical application of this compound:
Study | Population | Dosage | Results |
---|---|---|---|
Sakalis et al., 1974 | Psychiatric inpatients | Up to 200 mg/day | Significant reduction in anxiety for 8/10 patients; no side effects reported |
Sathananthan et al., 1976 | Patients with severe psychotic depression | Up to 200 mg/day | Significant improvement in 4/10 patients; non-responders did not benefit from standard antidepressants |
Q & A
Basic Research Questions
Q. What are the primary pharmacological targets of Cartazolate, and how can researchers design studies to validate these targets experimentally?
- Methodological Answer : To identify pharmacological targets, use a combination of in vitro binding assays (e.g., receptor affinity tests) and in silico molecular docking simulations. Ensure dose-response relationships are established across multiple concentrations. Validate findings using knockout models or competitive antagonists to confirm specificity . For experimental design, apply the PICOT framework (Population/Problem, Intervention, Comparison, Outcome, Time) to structure hypotheses, ensuring alignment with prior literature on GABAergic modulation .
Q. How should researchers select appropriate experimental models (e.g., in vitro vs. in vivo) for assessing this compound’s efficacy and safety?
- Methodological Answer : Prioritize in vitro models (e.g., cell lines expressing target receptors) for preliminary mechanistic studies, followed by in vivo models (rodents, non-human primates) for pharmacokinetic and behavioral analyses. Use stratified randomization to control for confounding variables like age or genetic background. Align model selection with the research question’s scope: narrow questions (e.g., receptor binding kinetics) may require only in vitro data, while broader questions (e.g., neurobehavioral effects) demand in vivo validation .
Advanced Research Questions
Q. How can researchers resolve contradictions in this compound’s reported efficacy across different experimental conditions?
- Methodological Answer : Conduct a meta-analysis of existing studies, categorizing variables such as dosage, administration route, and model systems. Use statistical tools (e.g., ANOVA or regression analysis) to identify confounding factors. For conflicting in vivo results, perform sensitivity analyses to assess the impact of sample size or measurement tools (e.g., behavioral assays vs. biomarkers). Cross-reference findings with structural analogs to isolate compound-specific effects .
Q. What methodological strategies optimize the reproducibility of this compound’s receptor-binding assays?
- Methodological Answer : Standardize protocols using guidelines from the Beilstein Journal of Organic Chemistry:
- Controls : Include positive (known agonists) and negative (vehicle-only) controls in each assay batch.
- Replication : Perform triplicate measurements across independent experimental runs.
- Data Reporting : Provide raw data (e.g., IC₅₀ values, binding curves) in supplementary materials with explicit descriptions of statistical thresholds (e.g., p < 0.01) .
Q. How can researchers design dose-response studies to minimize variability in this compound’s pharmacokinetic data?
- Methodological Answer : Implement a crossover study design to reduce inter-subject variability. Use pharmacokinetic modeling (e.g., non-compartmental analysis) to estimate AUC, Cₘₐₓ, and t₁/₂. Validate assays via LC-MS/MS for plasma concentration measurements, ensuring calibration curves meet FDA bioanalytical guidelines. Report variability metrics (e.g., coefficient of variation) for transparency .
Q. Data Analysis and Interpretation
Q. What statistical approaches are most robust for analyzing this compound’s neuroprotective effects in heterogeneous populations?
- Methodological Answer : Apply mixed-effects models to account for nested variables (e.g., genetic subgroups, sex differences). Use Bayesian inference to incorporate prior data on similar compounds, reducing Type I errors. For longitudinal data, employ survival analysis or repeated-measures ANOVA with Bonferroni correction .
Q. How should researchers address missing data in this compound’s clinical trial datasets?
- Methodological Answer : Apply multiple imputation techniques (e.g., MICE algorithm) for continuous variables, ensuring <10% missingness. For categorical data, use sensitivity analyses to compare complete-case vs. imputed results. Document all missing data patterns in supplementary materials to avoid bias .
Q. Experimental Design Tables
Properties
CAS No. |
34966-41-1 |
---|---|
Molecular Formula |
C15H22N4O2 |
Molecular Weight |
290.36 g/mol |
IUPAC Name |
ethyl 4-(butylamino)-1-ethylpyrazolo[3,4-b]pyridine-5-carboxylate |
InChI |
InChI=1S/C15H22N4O2/c1-4-7-8-16-13-11-10-18-19(5-2)14(11)17-9-12(13)15(20)21-6-3/h9-10H,4-8H2,1-3H3,(H,16,17) |
InChI Key |
IQNQAOGGWGCROX-UHFFFAOYSA-N |
SMILES |
CCCCNC1=C2C=NN(C2=NC=C1C(=O)OCC)CC |
Canonical SMILES |
CCCCNC1=C2C=NN(C2=NC=C1C(=O)OCC)CC |
Key on ui other cas no. |
34966-41-1 |
Synonyms |
cartazolate SQ 65,396 |
Origin of Product |
United States |
Synthesis routes and methods
Procedure details
Disclaimer and Information on In-Vitro Research Products
Please be aware that all articles and product information presented on BenchChem are intended solely for informational purposes. The products available for purchase on BenchChem are specifically designed for in-vitro studies, which are conducted outside of living organisms. In-vitro studies, derived from the Latin term "in glass," involve experiments performed in controlled laboratory settings using cells or tissues. It is important to note that these products are not categorized as medicines or drugs, and they have not received approval from the FDA for the prevention, treatment, or cure of any medical condition, ailment, or disease. We must emphasize that any form of bodily introduction of these products into humans or animals is strictly prohibited by law. It is essential to adhere to these guidelines to ensure compliance with legal and ethical standards in research and experimentation.