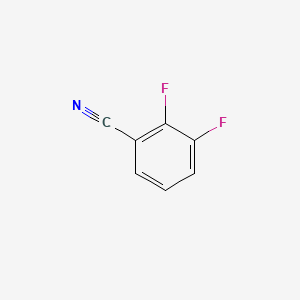
2,3-Difluorobenzonitrile
Overview
Description
2,3-Difluorobenzonitrile (CAS: 21524-39-0, molecular formula: C₇H₃F₂N, molecular weight: 139.10 g/mol) is a fluorinated aromatic nitrile characterized by two fluorine substituents at the ortho (2nd) and meta (3rd) positions of the benzene ring. Its structure confers unique electronic and steric properties, making it a valuable intermediate in organic synthesis, particularly in pharmaceuticals and agrochemicals. Key applications include its use in the preparation of macrocyclic compounds (e.g., aza-macrocyclic ligands) and as a precursor in transition-metal-free tandem reactions for synthesizing 1,4-benzodiazepine scaffolds .
Spectroscopic studies using Fourier transform microwave (FTMW) spectroscopy reveal its dipole moment components as μₐ = -3.39 D and μᵦ = -4.03 D, critical for understanding its rotational dynamics and molecular interactions . Quantum chemical calculations (MP2/6-311++G(2d,2p)) have provided insights into its equilibrium geometry, vibrational frequencies, and thermodynamic parameters (e.g., standard entropy and enthalpy changes) .
Preparation Methods
Fluorination of Chlorobenzonitriles Using Potassium Fluoride
Reaction Mechanism and Conditions
The fluorination of chlorobenzonitriles with potassium fluoride (KF) in aprotic polar solvents represents a well-established route for synthesizing fluorobenzonitriles. This method, detailed in EP0073372A1 , involves nucleophilic aromatic substitution (SNAr), where fluoride ions displace chlorine atoms under high-temperature conditions (100–250°C). The general reaction scheme is:
6\text{H}3\text{(CN)-Cl} + \text{KF} \xrightarrow{\text{solvent}} \text{F-C}6\text{H}3\text{(CN)-F} + \text{KCl}
Key parameters include:
-
Solvent : Sulfolane, dimethylformamide (DMF), or dimethyl sulfoxide (DMSO) enhance fluoride ion reactivity.
-
Temperature : 150–200°C optimizes substitution kinetics while minimizing side reactions.
-
Catalyst : Phase-transfer catalysts (e.g., 18-crown-6) improve fluoride availability in nonpolar media .
Table 1: Fluorination Conditions for Chlorobenzonitriles (Adapted from )
Starting Material | Solvent | Temperature (°C) | Time (h) | Yield (%) |
---|---|---|---|---|
2,3,6-Trichlorobenzonitrile | Sulfolane | 180 | 6 | 79.5 |
2,3,5,6-Tetrachlorobenzonitrile | DMF | 200 | 10 | 85 |
Application to 2,3-Difluorobenzonitrile
To synthesize this compound, 2,3-dichlorobenzonitrile would serve as the ideal precursor. Fluorination with KF in sulfolane at 180°C for 6 hours could theoretically yield the target compound. However, the patent emphasizes challenges in regioselectivity, as competing substitutions at adjacent positions may produce mixed isomers. Purification via recrystallization (e.g., using n-hexane) or distillation is critical to isolate the desired product.
Catalytic Hydrogenation of Chlorofluorobenzonitriles
Methodology and Catalytic Systems
EP0073372A1 describes the reduction of chlorofluorobenzonitriles (e.g., 3-chloro-2,6-difluorobenzonitrile) using hydrogen gas in the presence of palladium or platinum catalysts. The reaction proceeds via hydrodechlorination:
6\text{H}3\text{(CN)-F} + \text{H}2 \xrightarrow{\text{Pd/C}} \text{H-C}6\text{H}_3\text{(CN)-F} + \text{HCl}
Critical parameters include:
-
Catalyst : 5% Pd/C or PtO₂ at 0.5–2 wt% loading.
-
Temperature : 50–100°C to balance reaction rate and catalyst stability.
-
Pressure : 5–50 kg/cm² hydrogen pressure ensures sufficient reactant contact .
Table 2: Hydrogenation Conditions for Chlorofluorobenzonitriles (Data from )
Substrate | Catalyst | Pressure (kg/cm²) | Temperature (°C) | Yield (%) |
---|---|---|---|---|
3-Chloro-2,6-difluorobenzonitrile | Pd/C | 5 | 70 | 92 |
3,5-Dichloro-2,6-difluorobenzonitrile | Pt/C | 20 | 90 | 88 |
Relevance to this compound
For this compound synthesis, this method requires a precursor such as 3-chloro-2-fluorobenzonitrile. Hydrogenation under mild conditions (70°C, 5 kg/cm² H₂) with Pd/C could selectively remove the chlorine atom at position 3. However, the patent notes that over-reduction of fluorine atoms is a risk at temperatures >100°C, necessitating precise control.
Nitro-Group Substitution and Halex Reaction
Synthetic Pathway from Nitro Precursors
US5478963A outlines a route for 2,3-difluoro-6-nitrobenzonitrile synthesis, involving cyanide substitution on 2,3,4-trifluoronitrobenzene. Subsequent denitrating chlorination and halogen exchange (Halex reaction) yield fluorobenzonitriles:
Table 3: Reaction Steps and Conditions (Data from )
Step | Reagents/Conditions | Yield (%) |
---|---|---|
Cyanide Substitution | KCN in tert-butanol, 60°C, 4 h | 86 |
Denitrating Chlorination | Cl₂, 175–190°C, 2 h | 78 |
Halex Reaction | KF in sulfolane, 200°C, 4 h | 70 |
Adaptation for this compound
To target this compound, the nitro group in 2,3-difluoro-6-nitrobenzonitrile could be reduced to an amine (via catalytic hydrogenation) and subsequently removed via diazotization. For example:
2/\text{Pd-C}} \text{2,3-Difluoro-6-aminobenzonitrile} \xrightarrow{\text{NaNO}2/\text{HCl}} \text{this compound}
This pathway, while plausible, introduces complexity due to intermediate purification requirements and potential side reactions during diazotization .
Comparative Analysis of Synthetic Routes
Table 4: Advantages and Limitations of Each Method
Method | Advantages | Limitations |
---|---|---|
Fluorination with KF | High yields, scalable | Requires hazardous solvents (sulfolane) |
Catalytic Hydrogenation | Selective dehalogenation | Risk of over-reduction at high temperatures |
Nitro Substitution | Versatile for multi-step synthesis | Low overall yield due to multiple steps |
Industrial Considerations and Optimization
Catalyst Recovery and Recycling
Both patents highlight catalyst recovery via filtration (e.g., Pd/C) or distillation (triethylamine). For large-scale production, continuous flow systems could enhance efficiency, as demonstrated in EP0073372A1 , where a fluidized-bed reactor achieved 79.5% yield in 25-second residence time.
Chemical Reactions Analysis
Types of Reactions: 2,3-Difluorobenzonitrile undergoes various chemical reactions, including:
Nucleophilic Substitution: The fluorine atoms in this compound can be replaced by nucleophiles such as amines or thiols under appropriate conditions.
Reduction: The nitrile group can be reduced to an amine using reducing agents like lithium aluminum hydride (LiAlH4).
Oxidation: Although less common, the compound can undergo oxidation reactions, particularly at the nitrile group, to form carboxylic acids or other derivatives.
Common Reagents and Conditions:
Nucleophilic Substitution: Reagents like sodium amide (NaNH2) or potassium thiolate (KSR) are commonly used.
Reduction: Lithium aluminum hydride (LiAlH4) or catalytic hydrogenation are typical methods.
Oxidation: Strong oxidizing agents such as potassium permanganate (KMnO4) or chromium trioxide (CrO3) can be employed.
Major Products:
Substitution Reactions: Products include substituted benzonitriles with various functional groups.
Reduction Reactions: The primary product is 2,3-difluorobenzylamine.
Oxidation Reactions: Products can include 2,3-difluorobenzoic acid and related compounds.
Scientific Research Applications
Antimicrobial and Antiviral Properties
Recent studies have highlighted the antimicrobial and antiviral potential of fluorinated compounds, including 2,3-difluorobenzonitrile. Preliminary investigations suggest that these compounds may exhibit enhanced efficacy against various bacterial strains due to increased lipophilicity and metabolic stability attributed to the fluorine substituents.
- Case Study: Antiviral Activity
- Related compounds have shown promising results against HIV-1, with effective concentrations (EC50) ranging from 3 nM to over 100 nM.
Anticancer Potential
Fluorinated aromatic compounds like this compound are being explored for their anticancer properties. They may inhibit key metabolic enzymes in cancer cells, which could enhance selectivity and potency against specific cancer cell lines.
Pesticide Development
The compound serves as a precursor for synthesizing various agrochemicals. Notably, it is involved in producing pyrethroid pesticides and other plant protection agents through its conversion to 2,3,6-trifluorobenzoic acid, a crucial intermediate in pesticide formulation .
- Data Table: Synthesis Pathways for Pesticides
Compound | Intermediate | Application |
---|---|---|
This compound | 2,3,6-Trifluorobenzoic Acid | Pyrethroid Insecticides |
2-Chloro-5,6-difluorobenzonitrile | 2,3-Difluoro-6-nitrobenzonitrile | Antibacterial Agents |
Synthesis of Novel Materials
The compound has been utilized in synthesizing advanced materials such as difluorophenyl-1,2,3,5-dithiadiazolyl and cyanophenoxazines. These materials are explored for their electronic properties and potential applications in sensors and optoelectronic devices .
- Data Table: Material Applications of this compound
Material | Synthesis Method | Potential Application |
---|---|---|
Difluorophenyl Dithiadiazolyl | Reaction with thioketones | Sensors |
Cyanophenoxazines | Coupling reactions with phenols | Optoelectronic Devices |
Biocatalytic Processes
Recent advancements have leveraged biocatalytic processes using 6-amino-2,3-difluorobenzonitrile as a substrate to produce valuable intermediates like 2,6-difluorobenzamide. This process employs recombinant Escherichia coli expressing nitrile hydratase from Aurantimonas manganoxydans, resulting in high yields without by-product formation.
- Case Study: Biocatalytic Production
- Yield: 314 g/L of 2,6-difluorobenzamide produced within 11 hours.
Mechanism of Action
The mechanism by which 2,3-difluorobenzonitrile exerts its effects depends on the specific application. In chemical reactions, the fluorine atoms influence the reactivity of the benzene ring, making it more susceptible to nucleophilic attack. The nitrile group can participate in various transformations, contributing to the compound’s versatility in synthesis.
Molecular Targets and Pathways: In biological systems, the compound’s interactions with enzymes and receptors are of interest. The presence of fluorine atoms can enhance binding affinity and selectivity, making it a valuable tool in drug discovery and development.
Comparison with Similar Compounds
Comparison with Similar Difluorobenzonitrile Compounds
Difluorobenzonitriles exhibit distinct physicochemical and reactive properties depending on fluorine substitution patterns. Below is a comparative analysis of 2,3-difluorobenzonitrile with its isomers and related derivatives:
Structural and Electronic Properties
Compound | CAS Number | Fluorine Positions | Dipole Moments (D) | Melting Point (°C) | Key Applications |
---|---|---|---|---|---|
This compound | 21524-39-0 | 2,3-ortho/meta | μₐ = -3.39, μᵦ = -4.03 | Not reported | Pharmaceuticals, macrocycles |
2,4-Difluorobenzonitrile | 3939-09-1 | 2,4-ortho/para | μₐ = -4.13, μᵦ = -1.08 | 45–50 | Agrochemicals, herbicides |
3,4-Difluorobenzonitrile | 64248-62-0 | 3,4-meta/para | Not reported | 51–53 | Herbicide intermediates |
3,5-Difluorobenzonitrile | 64248-63-1 | 3,5-meta | Not reported | 84–86 | OLEDs, catalysts |
Key Observations:
- Dipole Moments : The ortho/meta substitution in 2,3-DFBN results in a higher μᵦ component compared to 2,4-DFBN (-4.03 D vs. -1.08 D), influencing solvation and intermolecular interactions .
- Thermodynamic Stability : 2,3-DFBN exhibits a higher activation energy (96.7 kJ·mol⁻¹) during hydrolysis compared to 2,6-DFBN (75.4 kJ·mol⁻¹), reflecting greater kinetic stability under aqueous conditions .
- Geometric Effects : Natural bond orbital (NBO) analysis indicates that ortho-fluorine substitution induces significant resonance-assisted hybridization changes, altering electron density at the nitrile group .
Market and Industrial Relevance
Biological Activity
2,3-Difluorobenzonitrile (DFBN), with the molecular formula C7H3F2N, is an organic compound that has garnered attention in various fields of research due to its potential biological activity. This article delves into the biochemical properties, mechanisms of action, and applications of DFBN, supported by relevant data and case studies.
Chemical Structure and Properties
DFBN is a derivative of benzonitrile where two hydrogen atoms on the benzene ring are substituted with fluorine atoms at the 2 and 3 positions. The presence of fluorine enhances the compound's lipophilicity and metabolic stability, which can significantly influence its biological interactions.
DFBN's biological activity is primarily attributed to its ability to interact with various biomolecules, including enzymes and receptors. The compound has been shown to play a role in:
- Enzyme Inhibition : DFBN can bind to specific enzymes, leading to either inhibition or activation of their activity. This interaction can alter metabolic pathways and cellular functions.
- Cell Signaling : It influences cell signaling pathways, potentially affecting gene expression and cellular metabolism. Studies suggest that DFBN can modulate the expression of genes involved in critical cellular processes.
2. Cellular Effects
Research indicates that DFBN affects various types of cells, leading to changes in cellular activities. For instance:
- Gene Expression : DFBN has been shown to alter the expression levels of specific genes in cellular models.
- Metabolic Pathways : The compound impacts metabolic pathways within cells, influencing overall cellular metabolism.
Case Studies and Research Findings
Several studies have documented the biological effects of DFBN:
- Study on Toxicity : A study investigating the dosage effects of DFBN in animal models revealed that lower doses may exhibit minimal effects while higher doses could lead to toxic outcomes. The threshold for significant biological activity was identified, emphasizing the importance of dosage in determining the compound's effects .
- Biochemical Pathway Analysis : In laboratory settings, DFBN was found to be relatively stable under standard conditions but exhibited varying effects depending on exposure duration. This stability is crucial for understanding its long-term impact on cellular functions .
Applications in Research and Industry
DFBN is being explored for various applications:
- Organic Synthesis : It serves as a building block for synthesizing more complex organic molecules, including pharmaceuticals and agrochemicals.
- Medicinal Chemistry : Ongoing research aims to evaluate its potential as a precursor for medicinal compounds due to its unique structural properties .
- Material Science : Its application extends to producing specialty chemicals and materials with unique properties .
Data Table: Summary of Biological Activities
Activity Type | Description | Findings/References |
---|---|---|
Enzyme Interaction | Binds to enzymes affecting activity | Alters metabolic pathways |
Gene Expression | Modulates expression levels | Significant changes observed |
Dosage Effects | Varies with dosage; lower doses minimal effects | Toxicity observed at higher doses |
Stability | Relatively stable under standard conditions | Effects vary with exposure duration |
Q & A
Basic Research Questions
Q. What are the established synthetic routes for 2,3-Difluorobenzonitrile in laboratory settings?
- Methodological Answer : The primary synthesis involves halogen-exchange fluorination of chlorinated precursors. For example, reacting 2,3-dichlorobenzonitrile with potassium fluoride (KF) in a polar aprotic solvent like 1,3-dimethylimidazolidin-2-one (DMI) at elevated temperatures (290°C) under pressure. Catalysts such as tetraphenylphosphonium bromide enhance fluorination efficiency . Purity is typically verified via gas chromatography (GC) and nuclear magnetic resonance (NMR) spectroscopy.
Q. Which spectroscopic techniques are critical for characterizing this compound?
- Methodological Answer : Key techniques include:
- <sup>19</sup>F and <sup>1</sup>H NMR : To confirm fluorine substitution patterns and aromatic proton environments.
- Infrared (IR) Spectroscopy : Identifies nitrile (C≡N) stretching vibrations (~2230 cm⁻¹) and C-F bonds.
- X-ray Crystallography : Resolves molecular geometry and crystal packing, critical for structure-activity studies .
Q. What solvents and reaction conditions are optimal for its synthesis?
- Methodological Answer : High-boiling solvents like DMI or sulfolane are preferred for halogen-exchange reactions due to their thermal stability. Reactions typically require temperatures >250°C and anhydrous conditions to prevent hydrolysis of intermediates. Catalytic amounts of phosphonium salts (e.g., tetraphenylphosphonium bromide) improve fluoride ion reactivity .
Advanced Research Questions
Q. How can researchers resolve contradictions in reported spectroscopic data for this compound?
- Methodological Answer : Discrepancies in <sup>19</sup>F NMR chemical shifts or IR bands may arise from solvent polarity or crystallographic variations. Cross-validation using isotopically labeled analogs (e.g., deuterated solvents for NMR) and computational modeling (DFT calculations) can clarify assignments. Referencing ground-state spectroscopic constants (e.g., rotational transitions) ensures accuracy .
Q. What strategies minimize by-product formation during halogen-exchange fluorination?
- Methodological Answer : By-products like monofluorinated or dichloro-fluorinated intermediates can be reduced by:
- Controlled stoichiometry : Excess KF (1.5–2.0 eq) drives complete fluorination.
- Catalyst optimization : Phosphonium salts at 5–10 mol% enhance reaction selectivity.
- Stepwise temperature ramping : Gradual heating avoids decomposition of thermally sensitive intermediates .
Q. How does the electronic influence of fluorine substituents affect reactivity in cross-coupling reactions?
- Methodological Answer : The meta -directing nature of fluorine alters regioselectivity in Suzuki-Miyaura or Ullmann couplings. Computational studies (e.g., Hammett σ constants) predict electron-withdrawing effects, which stabilize transition states in nucleophilic aromatic substitution. Experimental validation via kinetic isotope effects (KIEs) or substituent scrambling analysis is recommended .
Q. What are the hydrolysis kinetics of this compound under aqueous conditions?
- Methodological Answer : Hydrolysis studies in high-temperature water (HTW) reveal pseudo-first-order kinetics. Activation energy (Ea) and rate constants (k) are pH-dependent, with acidic conditions favoring nitrile conversion to carboxylic acids. Stability tests at 100–150°C inform storage protocols and reaction design .
Q. Applications in Academic Research
Q. How is this compound utilized in agrochemical intermediates?
- Methodological Answer : It serves as a precursor for fluorophenoxy herbicides. For example, nucleophilic displacement of the nitrile group with phenols yields diaryl ethers. Subsequent functionalization (e.g., esterification) generates bioactive molecules. Reaction yields are optimized via phase-transfer catalysis or microwave-assisted synthesis .
Q. What role does this compound play in polymer science?
- Methodological Answer : As a monomer in poly(arylene ether nitrile)s (PAENs), it enhances thermal stability and dielectric properties. Copolymerization with sulfonated monomers (e.g., potassium hydroquinonesulfonate) produces ion-exchange membranes for fuel cells. Molecular weight control is achieved via stoichiometric balancing of difluorobenzonitrile and bisphenol monomers .
Q. Can this compound act as a ligand in coordination chemistry?
- Methodological Answer : The nitrile group coordinates to transition metals (e.g., Pd, Cu) in catalytic cycles. Ligand design studies compare its binding affinity with para- or ortho-substituted analogs. X-ray absorption spectroscopy (XAS) and cyclic voltammetry (CV) characterize metal-ligand interactions .
Properties
IUPAC Name |
2,3-difluorobenzonitrile | |
---|---|---|
Source | PubChem | |
URL | https://pubchem.ncbi.nlm.nih.gov | |
Description | Data deposited in or computed by PubChem | |
InChI |
InChI=1S/C7H3F2N/c8-6-3-1-2-5(4-10)7(6)9/h1-3H | |
Source | PubChem | |
URL | https://pubchem.ncbi.nlm.nih.gov | |
Description | Data deposited in or computed by PubChem | |
InChI Key |
GKPHNZYMLJPYJJ-UHFFFAOYSA-N | |
Source | PubChem | |
URL | https://pubchem.ncbi.nlm.nih.gov | |
Description | Data deposited in or computed by PubChem | |
Canonical SMILES |
C1=CC(=C(C(=C1)F)F)C#N | |
Source | PubChem | |
URL | https://pubchem.ncbi.nlm.nih.gov | |
Description | Data deposited in or computed by PubChem | |
Molecular Formula |
C7H3F2N | |
Source | PubChem | |
URL | https://pubchem.ncbi.nlm.nih.gov | |
Description | Data deposited in or computed by PubChem | |
DSSTOX Substance ID |
DTXSID40175862 | |
Record name | 2,3-Difluorobenzonitrile | |
Source | EPA DSSTox | |
URL | https://comptox.epa.gov/dashboard/DTXSID40175862 | |
Description | DSSTox provides a high quality public chemistry resource for supporting improved predictive toxicology. | |
Molecular Weight |
139.10 g/mol | |
Source | PubChem | |
URL | https://pubchem.ncbi.nlm.nih.gov | |
Description | Data deposited in or computed by PubChem | |
CAS No. |
21524-39-0 | |
Record name | 2,3-Difluorobenzonitrile | |
Source | CAS Common Chemistry | |
URL | https://commonchemistry.cas.org/detail?cas_rn=21524-39-0 | |
Description | CAS Common Chemistry is an open community resource for accessing chemical information. Nearly 500,000 chemical substances from CAS REGISTRY cover areas of community interest, including common and frequently regulated chemicals, and those relevant to high school and undergraduate chemistry classes. This chemical information, curated by our expert scientists, is provided in alignment with our mission as a division of the American Chemical Society. | |
Explanation | The data from CAS Common Chemistry is provided under a CC-BY-NC 4.0 license, unless otherwise stated. | |
Record name | 2,3-Difluorobenzonitrile | |
Source | ChemIDplus | |
URL | https://pubchem.ncbi.nlm.nih.gov/substance/?source=chemidplus&sourceid=0021524390 | |
Description | ChemIDplus is a free, web search system that provides access to the structure and nomenclature authority files used for the identification of chemical substances cited in National Library of Medicine (NLM) databases, including the TOXNET system. | |
Record name | 2,3-Difluorobenzonitrile | |
Source | EPA DSSTox | |
URL | https://comptox.epa.gov/dashboard/DTXSID40175862 | |
Description | DSSTox provides a high quality public chemistry resource for supporting improved predictive toxicology. | |
Record name | 2,3-difluorobenzonitrile | |
Source | European Chemicals Agency (ECHA) | |
URL | https://echa.europa.eu/substance-information/-/substanceinfo/100.040.366 | |
Description | The European Chemicals Agency (ECHA) is an agency of the European Union which is the driving force among regulatory authorities in implementing the EU's groundbreaking chemicals legislation for the benefit of human health and the environment as well as for innovation and competitiveness. | |
Explanation | Use of the information, documents and data from the ECHA website is subject to the terms and conditions of this Legal Notice, and subject to other binding limitations provided for under applicable law, the information, documents and data made available on the ECHA website may be reproduced, distributed and/or used, totally or in part, for non-commercial purposes provided that ECHA is acknowledged as the source: "Source: European Chemicals Agency, http://echa.europa.eu/". Such acknowledgement must be included in each copy of the material. ECHA permits and encourages organisations and individuals to create links to the ECHA website under the following cumulative conditions: Links can only be made to webpages that provide a link to the Legal Notice page. | |
Retrosynthesis Analysis
AI-Powered Synthesis Planning: Our tool employs the Template_relevance Pistachio, Template_relevance Bkms_metabolic, Template_relevance Pistachio_ringbreaker, Template_relevance Reaxys, Template_relevance Reaxys_biocatalysis model, leveraging a vast database of chemical reactions to predict feasible synthetic routes.
One-Step Synthesis Focus: Specifically designed for one-step synthesis, it provides concise and direct routes for your target compounds, streamlining the synthesis process.
Accurate Predictions: Utilizing the extensive PISTACHIO, BKMS_METABOLIC, PISTACHIO_RINGBREAKER, REAXYS, REAXYS_BIOCATALYSIS database, our tool offers high-accuracy predictions, reflecting the latest in chemical research and data.
Strategy Settings
Precursor scoring | Relevance Heuristic |
---|---|
Min. plausibility | 0.01 |
Model | Template_relevance |
Template Set | Pistachio/Bkms_metabolic/Pistachio_ringbreaker/Reaxys/Reaxys_biocatalysis |
Top-N result to add to graph | 6 |
Feasible Synthetic Routes
Disclaimer and Information on In-Vitro Research Products
Please be aware that all articles and product information presented on BenchChem are intended solely for informational purposes. The products available for purchase on BenchChem are specifically designed for in-vitro studies, which are conducted outside of living organisms. In-vitro studies, derived from the Latin term "in glass," involve experiments performed in controlled laboratory settings using cells or tissues. It is important to note that these products are not categorized as medicines or drugs, and they have not received approval from the FDA for the prevention, treatment, or cure of any medical condition, ailment, or disease. We must emphasize that any form of bodily introduction of these products into humans or animals is strictly prohibited by law. It is essential to adhere to these guidelines to ensure compliance with legal and ethical standards in research and experimentation.