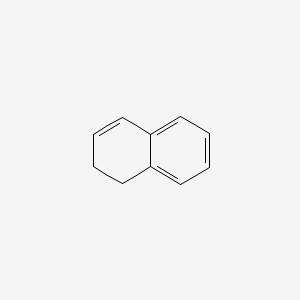
1,2-Dihydronaphthalene
Overview
Description
1,2-Dihydronaphthalene is a bicyclic hydrocarbon, which resembles naphthalene but shows partial unsaturation in one of its rings. Its derivatives find wide applications in natural compounds of therapeutic interest.
This compound is a dihydronaphthalene hydrogenated at C-1 and C-2.
Scientific Research Applications
Catalytic Asymmetric Construction
1,2-Dihydronaphthalenes are significant in both medicinal and synthetic chemistry. The oxidative N-heterocyclic carbene-catalyzed cascade annulation reactions using benzodiketones and enals under oxidative conditions are used to produce 1,2-dihydronaphthalenes. This process affords a variety of these compounds with high yield, diastereoselectivity, and enantioselectivity. The resulting products can be transformed into compounds such as alcohols, amides, and epoxides (Perveen et al., 2017).
Thermolysis Studies
The liquid-phase thermolysis of 1,2-dihydronaphthalene was studied in a batch reactor, showing that product distributions agree with calculations based on a free-radical scheme. This research aids in understanding the kinetic behavior of this compound under various conditions (Allen & Gavalas, 1983).
Thermodynamic Properties
Research on the thermodynamic properties of gaseous this compound includes methods like combustion-bomb calorimetry, adiabatic heat-capacity calorimetry, and densitometry. This research provides critical data on the standard molar entropies, enthalpies, and Gibbs free energies of formation, essential for understanding the compound's behavior under various conditions (Chirico & Steele, 2008).
NMR Spectroscopy Applications
This compound and its derivatives' n.m.r. spectra have been studied to understand their molecular structure and behavior. This research provides insights into the equilibrium and structural preferences of these molecules (Cook et al., 1969).
Infrared Spectroscopy and Astrophysics
This compound has been examined using infrared absorption spectroscopy and multiphoton infrared photodissociation action spectroscopy, contributing to a better understanding of the formation of H2 in the interstellar medium. This research highlights the role of this compound in astrophysical processes (Vala et al., 2009).
Electrolysis in Organic Synthesis
The electro-reduction of naphthalene, which produces this compound, has been explored in various solvents. This research is significant for understanding the selectivity and efficiency of this process in organic synthesis (Misono et al., 1968).
Photochemistry and Ring Contraction
The photochemistry of this compound oxide and its rearrangement to ring-contracted products like indan have been studied. This research is essential for understanding the photochemical behavior of this compound derivatives (White et al., 1993).
Mechanism of Action
Target of Action
1,2-Dihydronaphthalene primarily targets the naphthalene 1,2-dioxygenase enzyme . This enzyme is found in certain strains of bacteria, such as Pseudomonas putida . The enzyme plays a crucial role in the initial steps of naphthalene degradation .
Mode of Action
It is known that the enzyme catalyzes the incorporation of oxygen into naphthalene, a process that is essential for the degradation of this compound .
Biochemical Pathways
This compound is involved in the naphthalene catabolic pathway . The enzyme naphthalene 1,2-dioxygenase catalyzes the conversion of naphthalene to cis-1,2-dihydroxy-1,2-dihydronaphthalene . This is a key step in the degradation of naphthalene, which is an important process in certain bacteria that can utilize naphthalene as a source of carbon and energy .
Pharmacokinetics
It is known that the compound is a small molecule, which suggests that it may be readily absorbed and distributed in biological systems . The metabolism and excretion of this compound are likely to be influenced by the specific enzymes and transporters present in different organisms .
Result of Action
The action of this compound on its target enzyme results in the degradation of naphthalene, a polycyclic aromatic hydrocarbon . This process is beneficial for certain bacteria that can utilize naphthalene as a source of carbon and energy . The specific molecular and cellular effects of this compound’s action depend on the organism and the specific biochemical context .
Action Environment
The action, efficacy, and stability of this compound can be influenced by various environmental factors. For instance, the presence of naphthalene and the specific strains of bacteria can affect the activity of this compound . Additionally, factors such as temperature, pH, and the presence of other compounds can also influence the action of this compound .
Safety and Hazards
1,2-Dihydronaphthalene is a combustible liquid and causes serious eye irritation . It is advised to avoid dust formation, breathing mist, gas or vapours, and contact with skin and eye . Use personal protective equipment, wear chemical impermeable gloves, ensure adequate ventilation, remove all sources of ignition, and evacuate personnel to safe areas .
Biochemical Analysis
Biochemical Properties
1,2-Dihydronaphthalene plays a significant role in biochemical reactions, particularly in the context of oxidation processes. It is known to interact with enzymes such as naphthalene 1,2-dioxygenase, which catalyzes the initial oxidation of this compound to form cis-1,2-dihydroxy-1,2-dihydronaphthalene . This interaction is crucial as it represents the first step in the biodegradation pathway of naphthalene derivatives. Additionally, this compound can interact with other proteins and biomolecules involved in oxidative stress responses, potentially influencing cellular redox states.
Molecular Mechanism
At the molecular level, this compound exerts its effects through specific binding interactions with enzymes and other biomolecules. The compound’s interaction with naphthalene 1,2-dioxygenase involves the formation of a complex that facilitates the addition of oxygen atoms to the naphthalene ring, resulting in the production of dihydrodiol intermediates . This enzymatic activity is essential for the subsequent steps in the metabolic degradation of naphthalene derivatives. Additionally, this compound may act as an inhibitor or activator of other enzymes, depending on the cellular context and the presence of cofactors.
Metabolic Pathways
This compound is involved in metabolic pathways related to the degradation of aromatic hydrocarbons. The primary pathway involves its oxidation by naphthalene 1,2-dioxygenase to form cis-1,2-dihydroxy-1,2-dihydronaphthalene, which is further metabolized by other enzymes . This pathway is crucial for the detoxification and removal of naphthalene derivatives from the cellular environment. The interaction of this compound with specific cofactors and enzymes can influence metabolic flux and the levels of various metabolites.
Properties
IUPAC Name |
1,2-dihydronaphthalene | |
---|---|---|
Source | PubChem | |
URL | https://pubchem.ncbi.nlm.nih.gov | |
Description | Data deposited in or computed by PubChem | |
InChI |
InChI=1S/C10H10/c1-2-6-10-8-4-3-7-9(10)5-1/h1-3,5-7H,4,8H2 | |
Source | PubChem | |
URL | https://pubchem.ncbi.nlm.nih.gov | |
Description | Data deposited in or computed by PubChem | |
InChI Key |
KEIFWROAQVVDBN-UHFFFAOYSA-N | |
Source | PubChem | |
URL | https://pubchem.ncbi.nlm.nih.gov | |
Description | Data deposited in or computed by PubChem | |
Canonical SMILES |
C1CC2=CC=CC=C2C=C1 | |
Source | PubChem | |
URL | https://pubchem.ncbi.nlm.nih.gov | |
Description | Data deposited in or computed by PubChem | |
Molecular Formula |
C10H10 | |
Source | PubChem | |
URL | https://pubchem.ncbi.nlm.nih.gov | |
Description | Data deposited in or computed by PubChem | |
DSSTOX Substance ID |
DTXSID30858713 | |
Record name | 1,2-Dihydronaphthalene | |
Source | EPA DSSTox | |
URL | https://comptox.epa.gov/dashboard/DTXSID30858713 | |
Description | DSSTox provides a high quality public chemistry resource for supporting improved predictive toxicology. | |
Molecular Weight |
130.19 g/mol | |
Source | PubChem | |
URL | https://pubchem.ncbi.nlm.nih.gov | |
Description | Data deposited in or computed by PubChem | |
Physical Description |
Clear, faintly yellow-green, liquid; [MSDSonline] | |
Record name | 1,2-Dihydronaphthalene | |
Source | Haz-Map, Information on Hazardous Chemicals and Occupational Diseases | |
URL | https://haz-map.com/Agents/7902 | |
Description | Haz-Map® is an occupational health database designed for health and safety professionals and for consumers seeking information about the adverse effects of workplace exposures to chemical and biological agents. | |
Explanation | Copyright (c) 2022 Haz-Map(R). All rights reserved. Unless otherwise indicated, all materials from Haz-Map are copyrighted by Haz-Map(R). No part of these materials, either text or image may be used for any purpose other than for personal use. Therefore, reproduction, modification, storage in a retrieval system or retransmission, in any form or by any means, electronic, mechanical or otherwise, for reasons other than personal use, is strictly prohibited without prior written permission. | |
Vapor Pressure |
0.28 [mmHg] | |
Record name | 1,2-Dihydronaphthalene | |
Source | Haz-Map, Information on Hazardous Chemicals and Occupational Diseases | |
URL | https://haz-map.com/Agents/7902 | |
Description | Haz-Map® is an occupational health database designed for health and safety professionals and for consumers seeking information about the adverse effects of workplace exposures to chemical and biological agents. | |
Explanation | Copyright (c) 2022 Haz-Map(R). All rights reserved. Unless otherwise indicated, all materials from Haz-Map are copyrighted by Haz-Map(R). No part of these materials, either text or image may be used for any purpose other than for personal use. Therefore, reproduction, modification, storage in a retrieval system or retransmission, in any form or by any means, electronic, mechanical or otherwise, for reasons other than personal use, is strictly prohibited without prior written permission. | |
CAS No. |
447-53-0, 29828-28-2 | |
Record name | 1,2-Dihydronaphthalene | |
Source | CAS Common Chemistry | |
URL | https://commonchemistry.cas.org/detail?cas_rn=447-53-0 | |
Description | CAS Common Chemistry is an open community resource for accessing chemical information. Nearly 500,000 chemical substances from CAS REGISTRY cover areas of community interest, including common and frequently regulated chemicals, and those relevant to high school and undergraduate chemistry classes. This chemical information, curated by our expert scientists, is provided in alignment with our mission as a division of the American Chemical Society. | |
Explanation | The data from CAS Common Chemistry is provided under a CC-BY-NC 4.0 license, unless otherwise stated. | |
Record name | 1,2-Dihydronaphthalene | |
Source | ChemIDplus | |
URL | https://pubchem.ncbi.nlm.nih.gov/substance/?source=chemidplus&sourceid=0000447530 | |
Description | ChemIDplus is a free, web search system that provides access to the structure and nomenclature authority files used for the identification of chemical substances cited in National Library of Medicine (NLM) databases, including the TOXNET system. | |
Record name | Naphthalene, dihydro- | |
Source | ChemIDplus | |
URL | https://pubchem.ncbi.nlm.nih.gov/substance/?source=chemidplus&sourceid=0029828282 | |
Description | ChemIDplus is a free, web search system that provides access to the structure and nomenclature authority files used for the identification of chemical substances cited in National Library of Medicine (NLM) databases, including the TOXNET system. | |
Record name | 1,2-DIHYDRONAPHTHALENE | |
Source | DTP/NCI | |
URL | https://dtp.cancer.gov/dtpstandard/servlet/dwindex?searchtype=NSC&outputformat=html&searchlist=28463 | |
Description | The NCI Development Therapeutics Program (DTP) provides services and resources to the academic and private-sector research communities worldwide to facilitate the discovery and development of new cancer therapeutic agents. | |
Explanation | Unless otherwise indicated, all text within NCI products is free of copyright and may be reused without our permission. Credit the National Cancer Institute as the source. | |
Record name | 1,2-Dihydronaphthalene | |
Source | EPA DSSTox | |
URL | https://comptox.epa.gov/dashboard/DTXSID30858713 | |
Description | DSSTox provides a high quality public chemistry resource for supporting improved predictive toxicology. | |
Record name | 1,2-dihydronaphthalene | |
Source | European Chemicals Agency (ECHA) | |
URL | https://echa.europa.eu/substance-information/-/substanceinfo/100.006.532 | |
Description | The European Chemicals Agency (ECHA) is an agency of the European Union which is the driving force among regulatory authorities in implementing the EU's groundbreaking chemicals legislation for the benefit of human health and the environment as well as for innovation and competitiveness. | |
Explanation | Use of the information, documents and data from the ECHA website is subject to the terms and conditions of this Legal Notice, and subject to other binding limitations provided for under applicable law, the information, documents and data made available on the ECHA website may be reproduced, distributed and/or used, totally or in part, for non-commercial purposes provided that ECHA is acknowledged as the source: "Source: European Chemicals Agency, http://echa.europa.eu/". Such acknowledgement must be included in each copy of the material. ECHA permits and encourages organisations and individuals to create links to the ECHA website under the following cumulative conditions: Links can only be made to webpages that provide a link to the Legal Notice page. | |
Record name | 1,2-DIHYDRONAPHTHALENE | |
Source | FDA Global Substance Registration System (GSRS) | |
URL | https://gsrs.ncats.nih.gov/ginas/app/beta/substances/C765E5F653 | |
Description | The FDA Global Substance Registration System (GSRS) enables the efficient and accurate exchange of information on what substances are in regulated products. Instead of relying on names, which vary across regulatory domains, countries, and regions, the GSRS knowledge base makes it possible for substances to be defined by standardized, scientific descriptions. | |
Explanation | Unless otherwise noted, the contents of the FDA website (www.fda.gov), both text and graphics, are not copyrighted. They are in the public domain and may be republished, reprinted and otherwise used freely by anyone without the need to obtain permission from FDA. Credit to the U.S. Food and Drug Administration as the source is appreciated but not required. | |
Synthesis routes and methods I
Procedure details
Synthesis routes and methods II
Procedure details
Retrosynthesis Analysis
AI-Powered Synthesis Planning: Our tool employs the Template_relevance Pistachio, Template_relevance Bkms_metabolic, Template_relevance Pistachio_ringbreaker, Template_relevance Reaxys, Template_relevance Reaxys_biocatalysis model, leveraging a vast database of chemical reactions to predict feasible synthetic routes.
One-Step Synthesis Focus: Specifically designed for one-step synthesis, it provides concise and direct routes for your target compounds, streamlining the synthesis process.
Accurate Predictions: Utilizing the extensive PISTACHIO, BKMS_METABOLIC, PISTACHIO_RINGBREAKER, REAXYS, REAXYS_BIOCATALYSIS database, our tool offers high-accuracy predictions, reflecting the latest in chemical research and data.
Strategy Settings
Precursor scoring | Relevance Heuristic |
---|---|
Min. plausibility | 0.01 |
Model | Template_relevance |
Template Set | Pistachio/Bkms_metabolic/Pistachio_ringbreaker/Reaxys/Reaxys_biocatalysis |
Top-N result to add to graph | 6 |
Feasible Synthetic Routes
Disclaimer and Information on In-Vitro Research Products
Please be aware that all articles and product information presented on BenchChem are intended solely for informational purposes. The products available for purchase on BenchChem are specifically designed for in-vitro studies, which are conducted outside of living organisms. In-vitro studies, derived from the Latin term "in glass," involve experiments performed in controlled laboratory settings using cells or tissues. It is important to note that these products are not categorized as medicines or drugs, and they have not received approval from the FDA for the prevention, treatment, or cure of any medical condition, ailment, or disease. We must emphasize that any form of bodily introduction of these products into humans or animals is strictly prohibited by law. It is essential to adhere to these guidelines to ensure compliance with legal and ethical standards in research and experimentation.