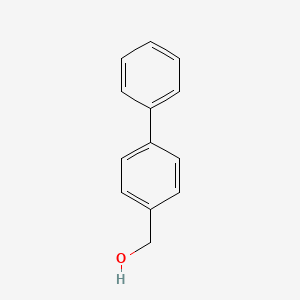
4-Biphenylmethanol
Overview
Description
4-Biphenylmethanol, also known as [1,1’-Biphenyl]-4-methanol, is an organic compound with the molecular formula C₁₃H₁₂O. It is characterized by a biphenyl structure with a hydroxymethyl group attached to the fourth carbon of one of the phenyl rings. This compound is a white to almost white crystalline solid and is known for its applications in various chemical processes .
Biochemical Analysis
Biochemical Properties
4-Biphenylmethanol plays a crucial role in biochemical reactions, particularly in the context of its interactions with enzymes and proteins. One notable interaction is with the cytochrome P450 monooxygenase enzyme CYP101B1, which efficiently and selectively oxidizes biphenyl derivatives . This interaction highlights the compound’s potential as a substrate in enzymatic reactions, where it undergoes oxidation to form various products. Additionally, this compound acts as a monofunctional alcohol initiator during the ring-opening polymerization of trimethylene carbonate, catalyzed by methanesulfonic acid .
Cellular Effects
This compound influences various cellular processes, including cell signaling pathways, gene expression, and cellular metabolism. Its impact on cell function is primarily mediated through its interactions with specific enzymes and proteins. For instance, the oxidation of this compound by CYP101B1 can lead to the generation of reactive oxygen species (ROS), which in turn can affect cellular signaling pathways and gene expression
Molecular Mechanism
The molecular mechanism of this compound involves its binding interactions with biomolecules, enzyme inhibition or activation, and changes in gene expression. The compound’s interaction with CYP101B1 results in the selective oxidation of its methyl group, leading to the formation of hydroxylated products Furthermore, this compound’s role as an initiator in polymerization reactions involves the activation of the hydroxymethyl group, which facilitates the ring-opening of trimethylene carbonate .
Temporal Effects in Laboratory Settings
In laboratory settings, the effects of this compound can change over time due to its stability and degradation. The compound is relatively stable under standard laboratory conditions, but its degradation can be influenced by factors such as temperature, pH, and the presence of other reactive species . Long-term studies have shown that this compound can maintain its activity in in vitro and in vivo settings, although its effects on cellular function may diminish over time due to gradual degradation and the formation of by-products.
Dosage Effects in Animal Models
The effects of this compound vary with different dosages in animal models. At low doses, the compound has been shown to have minimal toxic effects and can be used safely in biochemical studies . At higher doses, this compound can exhibit toxic or adverse effects, including oxidative stress and cellular damage. These threshold effects are crucial for determining the safe and effective dosage range for potential therapeutic applications.
Metabolic Pathways
This compound is involved in various metabolic pathways, including its oxidation by cytochrome P450 enzymes The compound’s metabolism involves the hydroxylation of its methyl group, leading to the formation of hydroxylated derivatives
Transport and Distribution
Within cells and tissues, this compound is transported and distributed through interactions with specific transporters and binding proteins. The compound’s hydrophobic nature allows it to diffuse across cell membranes, where it can interact with intracellular proteins and enzymes . Additionally, its distribution within tissues is influenced by factors such as blood flow, tissue permeability, and the presence of binding proteins that facilitate its transport and accumulation.
Subcellular Localization
The subcellular localization of this compound is primarily within the cytoplasm, where it interacts with various enzymes and proteins involved in metabolic processes The compound’s localization is influenced by targeting signals and post-translational modifications that direct it to specific cellular compartments
Preparation Methods
Synthetic Routes and Reaction Conditions: 4-Biphenylmethanol can be synthesized through several methods. One common method involves the reaction of biphenyl with paraformaldehyde in the presence of phosphoric acid and concentrated hydrochloric acid. The reaction is carried out at 100-120°C in a closed system for 2-48 hours. The mixture is then cooled, and the organic layer is separated and further processed to obtain the final product .
Industrial Production Methods: In industrial settings, the synthesis of this compound often involves the use of 4-biphenylcarboxylic acid as a starting material. This compound is first esterified with methanol or ethanol in the presence of sulfuric acid, followed by reduction with sodium borohydride or hydrogenation to yield this compound .
Chemical Reactions Analysis
Types of Reactions: 4-Biphenylmethanol undergoes various chemical reactions, including:
Oxidation: It can be oxidized to form 4-biphenylcarboxylic acid.
Reduction: Reduction of 4-biphenylcarboxylic acid can yield this compound.
Substitution: The hydroxyl group can be substituted with other functional groups under appropriate conditions
Common Reagents and Conditions:
Oxidation: Common oxidizing agents include potassium permanganate and chromium trioxide.
Reduction: Sodium borohydride and hydrogen gas in the presence of a catalyst are commonly used.
Substitution: Various reagents can be used depending on the desired substitution, such as halogenating agents for halogenation reactions
Major Products:
Oxidation: 4-Biphenylcarboxylic acid.
Reduction: this compound.
Substitution: Depending on the substituent, products can vary widely
Scientific Research Applications
4-Biphenylmethanol has a wide range of applications in scientific research:
Chemistry: It is used as a reagent in the synthesis of various organic compounds and as an initiator in polymerization reactions
Biology: It is used in studies involving cell transformation and as a model compound in biochemical research.
Medicine: It serves as an intermediate in the synthesis of pharmaceutical compounds.
Industry: It is used in the production of polymers and other industrial chemicals
Mechanism of Action
The mechanism of action of 4-Biphenylmethanol involves its role as an initiator in polymerization reactions. It acts by opening the ring of trimethylene carbonate, catalyzed by methanesulfonic acid, and copolymerizing with ε-caprolactone. This process is crucial in the formation of various polymers used in industrial applications .
Comparison with Similar Compounds
4-Biphenylmethanol can be compared with other similar compounds such as:
4-Phenylbenzyl alcohol: Similar structure but different functional group positioning.
4-Biphenylcarboxylic acid: Oxidized form of this compound.
4-Biphenylacetic acid: Contains an acetic acid group instead of a hydroxymethyl group
These compounds share structural similarities but differ in their functional groups and reactivity, making this compound unique in its applications and chemical behavior.
Properties
IUPAC Name |
(4-phenylphenyl)methanol | |
---|---|---|
Source | PubChem | |
URL | https://pubchem.ncbi.nlm.nih.gov | |
Description | Data deposited in or computed by PubChem | |
InChI |
InChI=1S/C13H12O/c14-10-11-6-8-13(9-7-11)12-4-2-1-3-5-12/h1-9,14H,10H2 | |
Source | PubChem | |
URL | https://pubchem.ncbi.nlm.nih.gov | |
Description | Data deposited in or computed by PubChem | |
InChI Key |
AXCHZLOJGKSWLV-UHFFFAOYSA-N | |
Source | PubChem | |
URL | https://pubchem.ncbi.nlm.nih.gov | |
Description | Data deposited in or computed by PubChem | |
Canonical SMILES |
C1=CC=C(C=C1)C2=CC=C(C=C2)CO | |
Source | PubChem | |
URL | https://pubchem.ncbi.nlm.nih.gov | |
Description | Data deposited in or computed by PubChem | |
Molecular Formula |
C13H12O | |
Source | PubChem | |
URL | https://pubchem.ncbi.nlm.nih.gov | |
Description | Data deposited in or computed by PubChem | |
DSSTOX Substance ID |
DTXSID5075234 | |
Record name | 4-Biphenylmethanol | |
Source | EPA DSSTox | |
URL | https://comptox.epa.gov/dashboard/DTXSID5075234 | |
Description | DSSTox provides a high quality public chemistry resource for supporting improved predictive toxicology. | |
Molecular Weight |
184.23 g/mol | |
Source | PubChem | |
URL | https://pubchem.ncbi.nlm.nih.gov | |
Description | Data deposited in or computed by PubChem | |
CAS No. |
3597-91-9 | |
Record name | [1,1′-Biphenyl]-4-methanol | |
Source | CAS Common Chemistry | |
URL | https://commonchemistry.cas.org/detail?cas_rn=3597-91-9 | |
Description | CAS Common Chemistry is an open community resource for accessing chemical information. Nearly 500,000 chemical substances from CAS REGISTRY cover areas of community interest, including common and frequently regulated chemicals, and those relevant to high school and undergraduate chemistry classes. This chemical information, curated by our expert scientists, is provided in alignment with our mission as a division of the American Chemical Society. | |
Explanation | The data from CAS Common Chemistry is provided under a CC-BY-NC 4.0 license, unless otherwise stated. | |
Record name | 4-Hydroxymethylbiphenyl | |
Source | ChemIDplus | |
URL | https://pubchem.ncbi.nlm.nih.gov/substance/?source=chemidplus&sourceid=0003597919 | |
Description | ChemIDplus is a free, web search system that provides access to the structure and nomenclature authority files used for the identification of chemical substances cited in National Library of Medicine (NLM) databases, including the TOXNET system. | |
Record name | 4-BIPHENYLMETHANOL | |
Source | DTP/NCI | |
URL | https://dtp.cancer.gov/dtpstandard/servlet/dwindex?searchtype=NSC&outputformat=html&searchlist=233860 | |
Description | The NCI Development Therapeutics Program (DTP) provides services and resources to the academic and private-sector research communities worldwide to facilitate the discovery and development of new cancer therapeutic agents. | |
Explanation | Unless otherwise indicated, all text within NCI products is free of copyright and may be reused without our permission. Credit the National Cancer Institute as the source. | |
Record name | 4-BIPHENYLMETHANOL | |
Source | DTP/NCI | |
URL | https://dtp.cancer.gov/dtpstandard/servlet/dwindex?searchtype=NSC&outputformat=html&searchlist=84169 | |
Description | The NCI Development Therapeutics Program (DTP) provides services and resources to the academic and private-sector research communities worldwide to facilitate the discovery and development of new cancer therapeutic agents. | |
Explanation | Unless otherwise indicated, all text within NCI products is free of copyright and may be reused without our permission. Credit the National Cancer Institute as the source. | |
Record name | 4-Biphenylmethanol | |
Source | EPA DSSTox | |
URL | https://comptox.epa.gov/dashboard/DTXSID5075234 | |
Description | DSSTox provides a high quality public chemistry resource for supporting improved predictive toxicology. | |
Record name | p-phenylbenzyl alcohol | |
Source | European Chemicals Agency (ECHA) | |
URL | https://echa.europa.eu/substance-information/-/substanceinfo/100.020.678 | |
Description | The European Chemicals Agency (ECHA) is an agency of the European Union which is the driving force among regulatory authorities in implementing the EU's groundbreaking chemicals legislation for the benefit of human health and the environment as well as for innovation and competitiveness. | |
Explanation | Use of the information, documents and data from the ECHA website is subject to the terms and conditions of this Legal Notice, and subject to other binding limitations provided for under applicable law, the information, documents and data made available on the ECHA website may be reproduced, distributed and/or used, totally or in part, for non-commercial purposes provided that ECHA is acknowledged as the source: "Source: European Chemicals Agency, http://echa.europa.eu/". Such acknowledgement must be included in each copy of the material. ECHA permits and encourages organisations and individuals to create links to the ECHA website under the following cumulative conditions: Links can only be made to webpages that provide a link to the Legal Notice page. | |
Record name | 4-BIPHENYLMETHANOL | |
Source | FDA Global Substance Registration System (GSRS) | |
URL | https://gsrs.ncats.nih.gov/ginas/app/beta/substances/GN8BN8XCF5 | |
Description | The FDA Global Substance Registration System (GSRS) enables the efficient and accurate exchange of information on what substances are in regulated products. Instead of relying on names, which vary across regulatory domains, countries, and regions, the GSRS knowledge base makes it possible for substances to be defined by standardized, scientific descriptions. | |
Explanation | Unless otherwise noted, the contents of the FDA website (www.fda.gov), both text and graphics, are not copyrighted. They are in the public domain and may be republished, reprinted and otherwise used freely by anyone without the need to obtain permission from FDA. Credit to the U.S. Food and Drug Administration as the source is appreciated but not required. | |
Synthesis routes and methods I
Procedure details
Synthesis routes and methods II
Procedure details
Synthesis routes and methods III
Procedure details
Synthesis routes and methods IV
Procedure details
Retrosynthesis Analysis
AI-Powered Synthesis Planning: Our tool employs the Template_relevance Pistachio, Template_relevance Bkms_metabolic, Template_relevance Pistachio_ringbreaker, Template_relevance Reaxys, Template_relevance Reaxys_biocatalysis model, leveraging a vast database of chemical reactions to predict feasible synthetic routes.
One-Step Synthesis Focus: Specifically designed for one-step synthesis, it provides concise and direct routes for your target compounds, streamlining the synthesis process.
Accurate Predictions: Utilizing the extensive PISTACHIO, BKMS_METABOLIC, PISTACHIO_RINGBREAKER, REAXYS, REAXYS_BIOCATALYSIS database, our tool offers high-accuracy predictions, reflecting the latest in chemical research and data.
Strategy Settings
Precursor scoring | Relevance Heuristic |
---|---|
Min. plausibility | 0.01 |
Model | Template_relevance |
Template Set | Pistachio/Bkms_metabolic/Pistachio_ringbreaker/Reaxys/Reaxys_biocatalysis |
Top-N result to add to graph | 6 |
Feasible Synthetic Routes
Disclaimer and Information on In-Vitro Research Products
Please be aware that all articles and product information presented on BenchChem are intended solely for informational purposes. The products available for purchase on BenchChem are specifically designed for in-vitro studies, which are conducted outside of living organisms. In-vitro studies, derived from the Latin term "in glass," involve experiments performed in controlled laboratory settings using cells or tissues. It is important to note that these products are not categorized as medicines or drugs, and they have not received approval from the FDA for the prevention, treatment, or cure of any medical condition, ailment, or disease. We must emphasize that any form of bodily introduction of these products into humans or animals is strictly prohibited by law. It is essential to adhere to these guidelines to ensure compliance with legal and ethical standards in research and experimentation.