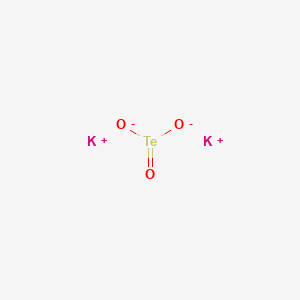
Potassium tellurite
- Click on QUICK INQUIRY to receive a quote from our team of experts.
- With the quality product at a COMPETITIVE price, you can focus more on your research.
Overview
Description
Potassium tellurite, with the chemical formula K₂TeO₃, is an inorganic compound composed of potassium and tellurium. It appears as a white crystalline powder and is known for its use in microbiological media due to its selective toxicity towards certain bacteria . This compound is particularly significant in the field of microbiology for differentiating bacterial species based on their ability to reduce tellurite to elemental tellurium, which results in black colonies.
Mechanism of Action
Target of Action
Potassium tellurite primarily targets the squalene monooxygenase enzyme , a key player in the cholesterol and ergosterol biosynthesis pathway, which is essential for cell wall synthesis in yeasts and molds . It also interacts with cytochrome c oxidase and NADH-dependent respiration pathways .
Mode of Action
This compound exerts its effects through the formation of superoxide radicals . This radical is derived, at least in part, from the enzymatic reduction of tellurite . The enzyme, tellurite reductase , reduces this compound, an electron acceptor, to metallic tellurite .
Biochemical Pathways
The toxicity of this compound in E. coli can be associated with the chemical activity of the tellurium oxyanion at various levels of bacterial metabolic pathways including, among others, inactivation of [Fe-S] center-containing dehydratases, cytoplasmic thiol oxidation, and enzyme .
Pharmacokinetics
It is known that this compound is a highly toxic compound for most microorganisms . Its toxicity is due to its ability to act as a strong oxidizing agent over a variety of cell components .
Result of Action
The result of this compound’s action is the generation of reactive oxygen species (ROS) . This leads to an increase in the generation of thiobarbituric acid-reactive substances (TBARs), inactivation of oxidative stress-sensitive [Fe-S] enzymes such as aconitase, and an increase of superoxide dismutase (SOD) activity .
Action Environment
The action of this compound is influenced by environmental factors. Its oxyanions are relatively stable environmentally, with tellurite being most abundant . The amount of tellurium released to the environment is increasing due to its applications in electronics, optics, batteries, and mining industries . This has led to an increase in environmental tellurium contamination, renewing biological interest in tellurium toxicity .
Biochemical Analysis
Biochemical Properties
Potassium tellurite plays a significant role in biochemical reactions, particularly in its interactions with enzymes, proteins, and other biomolecules. One of the primary interactions of this compound is with the enzyme superoxide dismutase (SOD). This compound induces the production of reactive oxygen species (ROS), which in turn activates SOD to mitigate oxidative stress . Additionally, this compound interacts with thiol-containing proteins, leading to the formation of tellurium-protein complexes that can disrupt normal cellular functions .
Cellular Effects
This compound has profound effects on various types of cells and cellular processes. In bacterial cells, this compound induces oxidative stress by generating reactive oxygen species, which can damage cellular components such as DNA, proteins, and lipids . This oxidative stress can lead to the activation of stress response pathways, including the upregulation of genes involved in antioxidant defense. In eukaryotic cells, this compound can interfere with cell signaling pathways, gene expression, and cellular metabolism, ultimately affecting cell viability and function .
Molecular Mechanism
The molecular mechanism of this compound involves its reduction to elemental tellurium within the cell. This reduction process generates reactive oxygen species, which can cause oxidative damage to cellular components . This compound also binds to thiol groups in proteins, leading to the formation of tellurium-protein complexes that can inhibit enzyme activity and disrupt normal cellular functions . Additionally, this compound can induce the expression of genes involved in oxidative stress response, further highlighting its impact on cellular processes .
Temporal Effects in Laboratory Settings
In laboratory settings, the effects of this compound can change over time due to its stability and degradation. This compound is relatively stable under standard laboratory conditions, but its effects on cellular function can vary depending on the duration of exposure. Long-term exposure to this compound can lead to the accumulation of oxidative damage and the activation of stress response pathways, which can have lasting effects on cellular function . In in vitro studies, the stability of this compound allows for consistent results over extended periods, making it a reliable tool for studying oxidative stress and microbial resistance .
Dosage Effects in Animal Models
The effects of this compound vary with different dosages in animal models. At low doses, this compound can induce mild oxidative stress and activate antioxidant defense mechanisms . At high doses, this compound can cause significant oxidative damage, leading to cell death and tissue damage . In animal models, threshold effects have been observed, where a certain dosage level triggers a pronounced toxic response. Additionally, high doses of this compound can result in toxic or adverse effects, including organ damage and impaired physiological functions .
Metabolic Pathways
This compound is involved in several metabolic pathways, particularly those related to oxidative stress and detoxification. The reduction of this compound to elemental tellurium is a key metabolic process that generates reactive oxygen species . This process involves enzymes such as superoxide dismutase and catalase, which help mitigate the oxidative stress induced by this compound . Additionally, this compound can affect metabolic flux and metabolite levels by disrupting normal cellular functions and inducing stress response pathways .
Transport and Distribution
Within cells and tissues, this compound is transported and distributed through various mechanisms. This compound can enter cells via passive diffusion or active transport mechanisms, depending on the cell type and environmental conditions . Once inside the cell, this compound can interact with transporters and binding proteins that facilitate its distribution to different cellular compartments . The localization and accumulation of this compound within cells can influence its activity and toxicity, with higher concentrations leading to more pronounced effects .
Subcellular Localization
The subcellular localization of this compound plays a crucial role in its activity and function. This compound can be found in various cellular compartments, including the cytoplasm, mitochondria, and nucleus . Its localization is influenced by targeting signals and post-translational modifications that direct it to specific organelles . In the cytoplasm, this compound can interact with thiol-containing proteins and enzymes, leading to the formation of tellurium-protein complexes . In the mitochondria, this compound can disrupt normal respiratory functions and induce oxidative stress, further highlighting its impact on cellular processes .
Preparation Methods
Synthetic Routes and Reaction Conditions: Potassium tellurite can be synthesized by reacting tellurium dioxide (TeO₂) with potassium hydroxide (KOH) in water. The reaction involves dissolving tellurium dioxide in water, followed by the addition of potassium hydroxide. The mixture is then heated and stirred until the reaction is complete, resulting in the formation of this compound. The solution is then filtered, and the filtrate is evaporated to obtain this compound crystals .
Industrial Production Methods: In industrial settings, this compound is produced by a similar method, but on a larger scale. The process involves the use of large reactors where tellurium dioxide and potassium hydroxide are mixed and heated. The resulting solution is then subjected to filtration and evaporation to yield this compound in bulk quantities .
Types of Reactions:
Reduction: this compound can be reduced to elemental tellurium by strong reducing agents or through electrolysis.
Oxidation: When fused with nitrate salts, this compound can be oxidized to potassium tellurate (K₂TeO₄).
Acidification: Acidifying aqueous solutions of this compound results in the precipitation of tellurium dioxide (TeO₂).
Common Reagents and Conditions:
Reducing Agents: Common reducing agents include hydrogen gas, sodium borohydride, and other strong reductants.
Oxidizing Agents: Nitrate salts are typically used for oxidation reactions.
Acidification: Strong acids like hydrochloric acid or sulfuric acid are used to acidify this compound solutions.
Major Products:
Elemental Tellurium (Te): Formed through reduction.
Potassium Tellurate (K₂TeO₄): Formed through oxidation.
Tellurium Dioxide (TeO₂): Formed through acidification.
Scientific Research Applications
Potassium tellurite has a wide range of applications in scientific research:
Microbiology: It is used in selective media to differentiate bacterial species based on their ability to reduce tellurite to tellurium.
Biochemistry: this compound is used to study oxidative stress and the mechanisms of resistance in bacteria.
Industry: It is used in the production of semiconductors and in the synthesis of other tellurium compounds.
Comparison with Similar Compounds
Sodium Tellurite (Na₂TeO₃): Similar in structure and reactivity but uses sodium instead of potassium.
Potassium Tellurate (K₂TeO₄): An oxidized form of potassium tellurite.
Sodium Tellurate (Na₂TeO₄): The sodium analog of potassium tellurate.
Uniqueness: this compound is unique due to its selective toxicity towards certain bacteria, making it valuable in microbiological applications. Its ability to generate ROS and induce oxidative stress is a distinctive feature that sets it apart from other tellurium compounds .
Properties
CAS No. |
7790-58-1 |
---|---|
Molecular Formula |
H2KO3Te |
Molecular Weight |
216.7 g/mol |
IUPAC Name |
dipotassium;tellurite |
InChI |
InChI=1S/K.H2O3Te/c;1-4(2)3/h;(H2,1,2,3) |
InChI Key |
FQRIUYARENIURW-UHFFFAOYSA-N |
SMILES |
[O-][Te](=O)[O-].[K+].[K+] |
Canonical SMILES |
O[Te](=O)O.[K] |
Key on ui other cas no. |
7790-58-1 |
Pictograms |
Acute Toxic; Irritant |
Synonyms |
potassium tellurate(IV) potassium tellurite |
Origin of Product |
United States |
Q1: What is potassium tellurite and what is its primary use in a research setting?
A: this compound (K2TeO3) is an inorganic compound commonly employed in microbiology research. It serves as a selective agent in culture media, inhibiting the growth of numerous Gram-negative bacteria while allowing the growth of certain Gram-positive bacteria, including specific pathogenic species. [, , , , , , , , , , , , , , ]
Q2: How does this compound exert its selective antimicrobial effect?
A: While the exact mechanism of tellurite's toxicity is still not fully understood, several hypotheses have been proposed. One prominent theory posits that this compound enters bacterial cells and undergoes reduction to metallic tellurium (Te0) within the cytoplasm. [, , , ] This reduction process is thought to be facilitated by intracellular oxidoreductases and may involve the utilization of NADH. [, ] The accumulation of insoluble tellurium crystals inside the bacterial cells is believed to be the primary cause of toxicity, potentially disrupting cellular processes and leading to cell death. [, ]
Q3: Are there specific bacterial species known to exhibit high resistance to this compound?
A: Yes, certain bacterial species, particularly some strains of Klebsiella pneumoniae and Escherichia coli O157:H7, are known to exhibit a high level of intrinsic resistance to this compound. [, , , , , ] This resistance is often associated with the presence of specific genes, such as the ter gene cluster, which encode for proteins involved in tellurite detoxification mechanisms. [, , , , ]
Q4: How can this resistance to this compound be beneficial in a research context?
A: The inherent resistance of certain bacterial species to this compound can be strategically exploited for their selective isolation and identification from clinical and environmental samples. For instance, incorporating this compound into MacConkey agar has proven effective in selectively culturing tellurite-resistant Klebsiella pneumoniae strains, particularly hypervirulent ones carrying the terW gene. [] This selective approach facilitates the detection and monitoring of potentially pathogenic bacteria in various settings. [, ]
Q5: Beyond its antimicrobial properties, does this compound have other applications in biological research?
A: Yes, this compound has shown potential as a tool for studying bacterial metabolism and respiratory activity. [] Research indicates that the reduction of tellurite by Mycobacterium leprae, although at low efficiency, provides a potential avenue to investigate the respiratory activities and metabolic pathways of this bacterium. []
Q6: What are the limitations of using this compound in bacterial research?
A: Despite its utility, this compound exhibits limitations. Some bacterial strains, including certain non-O157 Shiga toxin-producing Escherichia coli, display sensitivity to this compound, potentially leading to their underestimation in studies employing tellurite-containing selective media. [] Researchers must exercise caution and consider the potential impact of tellurite sensitivity when selecting appropriate media for specific bacterial isolation and enumeration.
Q7: Are there alternative selective agents that can be used in place of this compound?
A7: Yes, several alternative selective agents are available, with their choice depending on the specific research goals and the target bacterial species. Examples include:
- Sodium azide: A respiratory poison that inhibits cytochrome oxidase, often used to select for Gram-positive bacteria and suppress Gram-negative bacteria. [, ]
- Crystal violet: A dye that inhibits the growth of Gram-positive bacteria, frequently employed in selective media for Gram-negative bacteria. [, , ]
- Antibiotics: Various antibiotics, such as cefixime, cefsulodin, novobiocin, and bacitracin, can be incorporated into media to target specific bacterial groups or species. [, , ]
Disclaimer and Information on In-Vitro Research Products
Please be aware that all articles and product information presented on BenchChem are intended solely for informational purposes. The products available for purchase on BenchChem are specifically designed for in-vitro studies, which are conducted outside of living organisms. In-vitro studies, derived from the Latin term "in glass," involve experiments performed in controlled laboratory settings using cells or tissues. It is important to note that these products are not categorized as medicines or drugs, and they have not received approval from the FDA for the prevention, treatment, or cure of any medical condition, ailment, or disease. We must emphasize that any form of bodily introduction of these products into humans or animals is strictly prohibited by law. It is essential to adhere to these guidelines to ensure compliance with legal and ethical standards in research and experimentation.