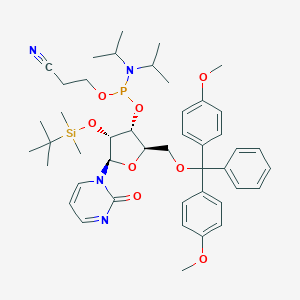
Zebularine CEP
Overview
Description
Mechanism of Action
Target of Action
Zebularine CEP, also known as 2/‘-O-T-BUTYLDIMETHYLSILYL-3/’-O-[(DIISOPROPYLAMINO)(2-CYANOETHOXY)PHOSPHINO]-5/‘-O-(4,4/’-DIMETHOXY, primarily targets cytidine deaminase . This enzyme plays a crucial role in the metabolism of cytidine, a nucleoside molecule that is integral to the structure of DNA .
Mode of Action
This compound acts as a transition state analog inhibitor of cytidine deaminase . It binds to the active site of the enzyme as covalent hydrates . This binding inhibits the enzyme’s activity, thereby affecting the metabolism of cytidine . Additionally, this compound has been shown to inhibit DNA methylation , a process that regulates gene expression .
Biochemical Pathways
The primary biochemical pathway affected by this compound is the DNA methylation pathway . By inhibiting DNA methylation, this compound can alter gene expression patterns . It has been suggested that this compound induces DNA-protein crosslinks (DPCs) of MET1 and zebularine-containing DNA . This could potentially affect various downstream cellular processes.
Result of Action
The inhibition of DNA methylation by this compound can lead to changes in gene expression. This can have various molecular and cellular effects, including the inhibition of tumor growth . In fact, this compound has been shown to display anti-tumor properties both in vitro and in vivo .
Biochemical Analysis
Biochemical Properties
Zebularine CEP acts as a transition state analog inhibitor of cytidine deaminase by binding to the active site as covalent hydrates . It is also known to inhibit DNA methylation, a process that involves the addition of a methyl group to the DNA molecule, which can significantly affect gene expression .
Cellular Effects
This compound has been shown to have various effects on cells. For instance, it has been found to protect against blood-brain-barrier (BBB) disruption in ischemic stroke (IS) models by increasing the expression of zona occludens-1 (ZO-1) and vascular endothelial (VE)-cadherin . It also demonstrated growth-inhibitory effects in cancer cells, occurring in parallel with its DNA demethylating action .
Molecular Mechanism
The molecular mechanism of this compound involves the formation of a covalent bond with DNA methyltransferases, leading to their inactivation . This results in a decrease in DNA methylation at selected loci, including a CACTA DNA transposon and a small region upstream from the UFGT gene, coding for the UDP glucose:flavonoid-3-O-glucosyltransferase .
Temporal Effects in Laboratory Settings
In laboratory settings, this compound has been shown to have long-term effects. For instance, in a mouse model of radiation-induced lymphomagenesis, 40% of this compound-treated animals were still alive after 1 year . Moreover, this compound treatment led to increased levels of interferon response in a cyclic guanosine monophosphate-AMP (cGAMP) synthase (cGAS)- and stimulator of interferon genes (STING)-dependent manner .
Dosage Effects in Animal Models
In animal models, the effects of this compound have been shown to vary with different dosages. For example, in a study on ear pinna wound closure in mice, this compound treatment resulted in improved ear hole repair .
Metabolic Pathways
This compound is involved in the metabolic pathway of DNA methylation, where it acts as an inhibitor of DNA methyltransferases . This results in a decrease in DNA methylation, thereby affecting gene expression .
Subcellular Localization
Given its role as a DNA methyltransferase inhibitor, it is likely that it localizes to the nucleus where DNA methylation occurs .
Preparation Methods
Synthetic Routes and Reaction Conditions: Zebularine can be synthesized through the condensation of 2-pyrimidinone with ribose derivatives. The reaction typically involves the use of acidic catalysts to facilitate the formation of the glycosidic bond . The process requires careful control of temperature and pH to ensure high yield and purity.
Industrial Production Methods: Industrial production of zebularine involves large-scale synthesis using optimized reaction conditions to maximize yield and minimize impurities. The process includes purification steps such as crystallization and chromatography to obtain zebularine in its pure form .
Chemical Reactions Analysis
Types of Reactions: Zebularine undergoes various chemical reactions, including:
Oxidation: Zebularine can be oxidized to form reactive intermediates that interact with DNA.
Substitution: It can participate in nucleophilic substitution reactions, particularly at the ribose moiety.
Common Reagents and Conditions:
Oxidizing Agents: Hydrogen peroxide and other peroxides are commonly used.
Nucleophiles: Ammonia and amines are typical nucleophiles in substitution reactions.
Major Products: The major products formed from these reactions include modified nucleosides and DNA adducts, which are crucial for studying DNA methylation and gene expression .
Scientific Research Applications
Zebularine has a wide range of applications in scientific research:
Chemistry: Used as a tool to study DNA methylation and its effects on gene expression.
Biology: Employed in epigenetic studies to understand the regulation of gene activity.
Industry: Utilized in the development of epigenetic drugs and diagnostic tools.
Comparison with Similar Compounds
- 5-Azacytidine
- 2-Deoxy-5-azacytidine
Comparison: Zebularine is unique due to its chemical stability and lower toxicity compared to other DNA methylation inhibitors like 5-azacytidine and 2-deoxy-5-azacytidine . While all three compounds inhibit DNA methylation, zebularine’s stability makes it more suitable for long-term studies and therapeutic applications .
Properties
IUPAC Name |
3-[[(2R,3R,4R,5R)-2-[[bis(4-methoxyphenyl)-phenylmethoxy]methyl]-4-[tert-butyl(dimethyl)silyl]oxy-5-(2-oxopyrimidin-1-yl)oxolan-3-yl]oxy-[di(propan-2-yl)amino]phosphanyl]oxypropanenitrile | |
---|---|---|
Source | PubChem | |
URL | https://pubchem.ncbi.nlm.nih.gov | |
Description | Data deposited in or computed by PubChem | |
InChI |
InChI=1S/C45H61N4O8PSi/c1-32(2)49(33(3)4)58(54-30-15-27-46)56-40-39(55-42(48-29-16-28-47-43(48)50)41(40)57-59(10,11)44(5,6)7)31-53-45(34-17-13-12-14-18-34,35-19-23-37(51-8)24-20-35)36-21-25-38(52-9)26-22-36/h12-14,16-26,28-29,32-33,39-42H,15,30-31H2,1-11H3/t39-,40-,41-,42-,58?/m1/s1 | |
Source | PubChem | |
URL | https://pubchem.ncbi.nlm.nih.gov | |
Description | Data deposited in or computed by PubChem | |
InChI Key |
UEUDLVPTCOPGMZ-MGVQLQBBSA-N | |
Source | PubChem | |
URL | https://pubchem.ncbi.nlm.nih.gov | |
Description | Data deposited in or computed by PubChem | |
Canonical SMILES |
CC(C)N(C(C)C)P(OCCC#N)OC1C(OC(C1O[Si](C)(C)C(C)(C)C)N2C=CC=NC2=O)COC(C3=CC=CC=C3)(C4=CC=C(C=C4)OC)C5=CC=C(C=C5)OC | |
Source | PubChem | |
URL | https://pubchem.ncbi.nlm.nih.gov | |
Description | Data deposited in or computed by PubChem | |
Isomeric SMILES |
CC(C)N(C(C)C)P(OCCC#N)O[C@@H]1[C@H](O[C@H]([C@@H]1O[Si](C)(C)C(C)(C)C)N2C=CC=NC2=O)COC(C3=CC=CC=C3)(C4=CC=C(C=C4)OC)C5=CC=C(C=C5)OC | |
Source | PubChem | |
URL | https://pubchem.ncbi.nlm.nih.gov | |
Description | Data deposited in or computed by PubChem | |
Molecular Formula |
C45H61N4O8PSi | |
Source | PubChem | |
URL | https://pubchem.ncbi.nlm.nih.gov | |
Description | Data deposited in or computed by PubChem | |
Molecular Weight |
845.0 g/mol | |
Source | PubChem | |
URL | https://pubchem.ncbi.nlm.nih.gov | |
Description | Data deposited in or computed by PubChem | |
Retrosynthesis Analysis
AI-Powered Synthesis Planning: Our tool employs the Template_relevance Pistachio, Template_relevance Bkms_metabolic, Template_relevance Pistachio_ringbreaker, Template_relevance Reaxys, Template_relevance Reaxys_biocatalysis model, leveraging a vast database of chemical reactions to predict feasible synthetic routes.
One-Step Synthesis Focus: Specifically designed for one-step synthesis, it provides concise and direct routes for your target compounds, streamlining the synthesis process.
Accurate Predictions: Utilizing the extensive PISTACHIO, BKMS_METABOLIC, PISTACHIO_RINGBREAKER, REAXYS, REAXYS_BIOCATALYSIS database, our tool offers high-accuracy predictions, reflecting the latest in chemical research and data.
Strategy Settings
Precursor scoring | Relevance Heuristic |
---|---|
Min. plausibility | 0.01 |
Model | Template_relevance |
Template Set | Pistachio/Bkms_metabolic/Pistachio_ringbreaker/Reaxys/Reaxys_biocatalysis |
Top-N result to add to graph | 6 |
Feasible Synthetic Routes
Disclaimer and Information on In-Vitro Research Products
Please be aware that all articles and product information presented on BenchChem are intended solely for informational purposes. The products available for purchase on BenchChem are specifically designed for in-vitro studies, which are conducted outside of living organisms. In-vitro studies, derived from the Latin term "in glass," involve experiments performed in controlled laboratory settings using cells or tissues. It is important to note that these products are not categorized as medicines or drugs, and they have not received approval from the FDA for the prevention, treatment, or cure of any medical condition, ailment, or disease. We must emphasize that any form of bodily introduction of these products into humans or animals is strictly prohibited by law. It is essential to adhere to these guidelines to ensure compliance with legal and ethical standards in research and experimentation.