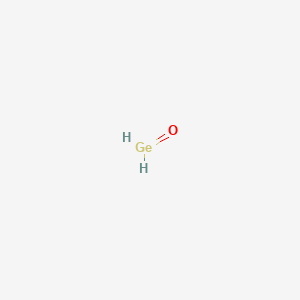
Germanium monoxide
Overview
Description
Germanium monoxide (GeO) is a subvalent germanium oxide with the chemical formula GeO. It exists as a metastable compound, typically forming as an intermediate during the oxidation of elemental germanium (Ge) to germanium dioxide (GeO₂) . Structurally, GeO is a dark, needle-like crystalline solid that sublimes at 710°C . Its metastability is evident from its tendency to oxidize further upon heating in air, ultimately converting to GeO₂ .
GeO is synthesized through non-adiabatic reaction dynamics in the gas phase, involving intersystem crossing (ISC) from a singlet collision complex (GeOO) to yield GeO and atomic oxygen . This method contrasts with the thermal oxidation of Ge substrates, where residual oxygen in annealing environments facilitates GeO formation before GeO₂ growth .
Preparation Methods
Gas-Phase Synthesis via Non-Adiabatic Reaction Dynamics
Reaction Mechanism and Electronic Surface Crossing
The gas-phase preparation of germanium monoxide involves the reaction of ground-state germanium atoms (Ge, ) with molecular oxygen (O, ) under single-collision conditions . This process proceeds through a multistep mechanism:
-
Barrierless Addition : Ge () reacts with O () on the singlet electronic surface, forming a linear GeOO intermediate (, , ) without an activation energy barrier .
-
Intersystem Crossing (ISC) : The singlet GeOO intermediate undergoes ISC to the triplet surface, facilitated by the "heavy atom effect" of germanium, which enhances spin-orbit coupling .
-
Dissociation : The triplet GeOO* complex decomposes into GeO () and atomic oxygen (O, ) .
The reaction is highly exothermic (), with 49% of the energy partitioned into translational motion of the products .
Table 1: Key Parameters of Gas-Phase GeO Synthesis
Parameter | Value | Reference |
---|---|---|
Reactants | Ge () + O () | |
Intermediate Geometry | Linear GeOO () | |
ISC Efficiency | >95% | |
Energy Partitioning | 49% translational | |
Product State Distribution | GeO () + O () |
Experimental Validation via Crossed Molecular Beams
Crossed beam experiments coupled with velocity map imaging confirm the dominance of the singlet GeO product :
-
Detection Methods : Rotational-resolved laser-induced fluorescence (LIF) and time-of-flight mass spectrometry identify GeO () with vibrational quantum numbers .
-
Angular Distribution : Forward-backward symmetric scattering patterns indicate a long-lived GeOO complex prior to dissociation .
-
Branching Ratio : >98% of product flux channels into GeO () rather than triplet GeO () .
Computational Insights into Reaction Pathways
Comparative Analysis of Synthetic Methods
Table 2: Method Comparison for GeO Preparation
Method | Yield | Purity | Energy Input | Scalability |
---|---|---|---|---|
Gas-Phase (Ge + O) | >95% | Ultrahigh | 418 kJ/mol | Lab-scale |
Solid-State Reduction | N/A | Unknown | High | Unproven |
The gas-phase method offers superior control over product states but requires advanced vacuum instrumentation. Solid-state approaches, while potentially scalable, lack mechanistic validation in peer-reviewed literature.
Chemical Reactions Analysis
Formation Reactions
Germanium monoxide can be synthesized through several pathways:
Gas-Phase Synthesis
GeO forms via the reaction of ground-state germanium atoms (Ge, ) with molecular oxygen (O, ) under single-collision conditions . This process involves:
-
Barrierless addition of Ge to O on the singlet surface, forming a linear GeOO intermediate (, ).
-
Intersystem crossing (ISC) to the triplet surface, yielding GeO () and ground-state oxygen atoms [O()] .
-
The reaction is exothermic () , with 49% of energy channeled into translational motion of products .
Solid-State Synthesis
Heating germanium dioxide (GeO) with elemental germanium at 1000°C produces GeO as a yellow sublimate :
Table 1: Key Formation Pathways
Reaction | Conditions | Products | Energy () | References |
---|---|---|---|---|
Ge + O | Gas phase, single-collision | GeO + O() | ||
GeO + Ge | 1000°C, solid state | 2 GeO | N/A |
Thermal Decomposition
GeO decomposes at elevated temperatures (≈650°C) into germanium and germanium dioxide :
his disproportionation highlights GeO’s instability under thermal stress.
Table 2: Thermal Decomposition Parameters
Reaction | Temperature | Products | Reference |
---|---|---|---|
2 GeO → Ge + GeO | 650°C | Ge, GeO |
Redox Reactions
Oxidation
GeO oxidizes to GeO when heated in oxygen :
Reduction*
GeO can be reduced to elemental germanium using hydrogen or carbon monoxide :
Table 3: Oxidation and Reduction Pathways
Reaction | Conditions | Products | Reference |
---|---|---|---|
GeO + O → GeO | Heating in O | GeO | |
GeO + H → Ge | Reduction with |
Scientific Research Applications
Optoelectronic Applications
Germanium monoxide exhibits significant potential in the field of optoelectronics due to its semiconducting properties. Its applications include:
- Photodetectors : GeO is utilized in the fabrication of photodetectors, which are critical components in optical communication systems. The compound's ability to absorb light and convert it into electrical signals makes it ideal for this purpose.
- Light Emitting Devices : Research indicates that this compound can be integrated into light-emitting devices, where it can help improve efficiency and performance due to its favorable electronic structure.
- Thin Film Transistors : GeO is also explored for use in thin film transistors, essential for display technologies and flexible electronics. The deposition of GeO thin films through techniques like atomic layer deposition allows for precise control over film thickness and composition.
Energy Storage
This compound is being investigated for its role in energy storage technologies:
- Lithium-Ion Batteries : As an anode material, this compound demonstrates high theoretical capacity, making it a promising candidate for next-generation lithium-ion batteries. Studies show that incorporating GeO can enhance the overall energy density and cycling stability of these batteries .
Catalytic Applications
The catalytic properties of this compound are being explored in various chemical reactions:
- Catalysis : GeO serves as a catalyst in several organic reactions, enhancing reaction rates and selectivity. Its amphoteric nature allows it to interact effectively with both acids and bases, making it versatile for different catalytic processes.
Research into the biological implications of this compound has revealed several potential therapeutic applications:
- Immunomodulation : Germanium compounds, including GeO, have been shown to enhance immune responses by increasing interferon production and modulating cytokine levels. These effects suggest potential applications in immunotherapy .
- Anticancer Activity : Studies indicate that germanium compounds may possess anticancer properties by normalizing cellular respiration and enhancing oxidative phosphorylation. This mechanism could retard tumor growth, making this compound a candidate for cancer treatment research .
Material Science
In material science, this compound's unique structural properties are being harnessed:
- Nanostructured Materials : The formation of nanocrystals from GeO has been investigated for applications in photonics and electronics. Controlled synthesis methods allow for the creation of germanium nanocrystals with tailored optical properties .
Summary Table of Applications
Application Area | Specific Uses | Benefits/Properties |
---|---|---|
Optoelectronics | Photodetectors, Light Emitting Devices | High absorption efficiency |
Energy Storage | Lithium-Ion Battery Anodes | Increased energy density |
Catalysis | Various Organic Reactions | Versatile catalytic activity |
Biological Activity | Immunomodulation, Anticancer Research | Enhances immune response |
Material Science | Nanostructured Materials | Tailored optical properties |
Mechanism of Action
The mechanism of action of germanium oxide involves its interaction with molecular targets and pathways:
Semiconducting Properties: Germanium oxide exhibits semiconducting properties, which are utilized in electronic and optoelectronic applications.
Amphoteric Nature: Its ability to react with both acids and bases makes it versatile in various chemical processes.
Optical Properties: Germanium oxide has unique optical properties, including high transparency and strong light-matter interaction, making it suitable for optoelectronic devices.
Comparison with Similar Compounds
Germanium Dioxide (GeO₂)
GeO₂ is the fully oxidized form of germanium, with two polymorphs (tetragonal and hexagonal) exhibiting higher thermal stability than GeO. Unlike GeO, GeO₂ is non-reactive in air and serves as a precursor for germanium-based nanomaterials in biomedical research .
Germanium Sesquioxide (Ge₂O₃)
Ge₂O₃, or germanium(III) oxide, is less studied but noted for its controversial biological applications. While early studies suggested anticancer properties, subsequent research highlighted methodological errors and misrepresentations in these claims . In contrast, GeO’s applications are primarily material science-oriented, such as in laser-treated heterolayers for nanofoam production .
Carbon Monoxide (CO)
CO is a diatomic gas with distinct toxicological profiles and detection methods. While GeO is analyzed via electrochemical techniques like stripping polarography , CO poisoning is diagnosed using carboxyhemoglobin measurements .
Silicon Monoxide (SiO)
However, SiO is more refractory (sublimes at ~1,100°C) and is used in optical coatings, whereas GeO’s lower sublimation temperature limits its analogous applications .
Biological Activity
Germanium monoxide (GeO) is a compound that has garnered attention for its potential biological activities, particularly in the fields of immunology and antioxidant defense. This article synthesizes current research findings on the biological activity of GeO, highlighting its mechanisms, effects on various biological systems, and potential therapeutic applications.
Overview of this compound
This compound is a subvalent germanium compound, primarily studied for its unique electronic properties and potential applications in materials science. However, its biological implications are increasingly being explored. GeO is formed through various chemical processes, often involving the reaction of germanium with oxygen under specific conditions .
Immune Modulation
Research indicates that germanium compounds, including GeO, can enhance immune responses. Key findings include:
- Interferon Production : Germanium compounds such as Ge-132 have been shown to significantly increase interferon levels in serum after administration in animal models. This enhancement plays a crucial role in activating natural killer (NK) cells and macrophages, which are vital components of the immune system .
- Cytokine Regulation : GeO may inhibit the secretion of pro-inflammatory cytokines such as TNF-α, IL-1β, and IL-6 by modulating pathways like nuclear factor-kappa B (NF-κB) and mitogen-activated protein kinase (MAPK) .
Antioxidant Activity
GeO exhibits notable antioxidant properties:
- Reactive Oxygen Species Scavenging : Germanium compounds can scavenge reactive oxygen species (ROS), thereby reducing oxidative stress. This activity is particularly important in preventing cellular damage associated with aging and various diseases .
- Enzymatic Activity Enhancement : Studies have reported that GeO can enhance the activity of antioxidant enzymes such as glutathione peroxidase (GSH-Px) and superoxide dismutase (SOD), contributing to a reduced level of lipid peroxidation in tissues .
Animal Studies
- Immune Response in Mice : In a study involving mice administered with Ge-132, significant increases in NK cell activity and macrophage activation were observed, correlating with enhanced interferon production .
- Oxidative Stress Models : In models subjected to oxidative stress, germanium compounds demonstrated protective effects against cellular damage by reducing hydrogen peroxide levels .
Human Studies
- Chronic Toxicity Cases : While exploring the therapeutic potential, it is crucial to note the adverse effects associated with prolonged exposure to germanium compounds. Reports indicate cases of chronic germanium intoxication leading to renal failure and neurological symptoms in patients who ingested high doses over extended periods .
- Supplementation Hazards : The consumption of germanium supplements has been linked to serious health risks, including nephrotoxicity. A review highlighted at least 31 cases of renal dysfunction associated with long-term intake of germanium products .
Data Summary
The following table summarizes key findings related to the biological activity of this compound:
Q & A
Q. Basic: What experimental protocols are recommended for synthesizing high-purity germanium monoxide (GeO) under controlled conditions?
Methodological Answer:
GeO synthesis typically involves controlled oxidation/reduction reactions of germanium precursors. For lab-scale preparation:
- Reduction of GeO₂ : Use hydrogen gas (H₂) at 600–700°C to reduce GeO₂ to GeO, monitoring oxygen partial pressure to avoid over-reduction to elemental Ge .
- Vapor Deposition : Sublimate GeO in a vacuum chamber at 900–1000°C, followed by controlled condensation to minimize impurities .
- Key Validation : Characterize purity via X-ray diffraction (XRD) for crystallinity and inductively coupled plasma mass spectrometry (ICP-MS) for trace contaminants. Report deviations in yield or impurity profiles, referencing established protocols in supplementary materials .
Q. Basic: How should researchers address discrepancies between theoretical and experimental bandgap values for GeO?
Methodological Answer:
Bandgap inconsistencies often arise from sample purity or measurement techniques. To resolve:
- Experimental Calibration : Use UV-Vis spectroscopy with a calibrated standard (e.g., silicon reference samples) and report atmospheric conditions (humidity/O₂ levels), as GeO is hygroscopic .
- Computational Cross-Check : Compare density functional theory (DFT) simulations (e.g., using VASP or Gaussian) with experimental data. Adjust for spin-orbit coupling and exchange-correlation functionals (e.g., PBE vs. HSE06) to match observed results .
- Data Transparency : Document raw spectra and computational parameters in FAIR-aligned repositories for peer validation .
Q. Advanced: What strategies optimize GeO thin-film stability for optoelectronic applications under ambient conditions?
Methodological Answer:
GeO degrades via oxidation and moisture absorption. Mitigation approaches:
- Encapsulation Layers : Deposit Al₂O₃ or SiO₂ via atomic layer deposition (ALD) to create moisture barriers. Quantify effectiveness using ellipsometry and accelerated aging tests (85°C/85% RH) .
- Doping Techniques : Introduce nitrogen (N₂) during sputtering to form GeON alloys, enhancing oxidative stability. Characterize using X-ray photoelectron spectroscopy (XPS) to confirm N incorporation .
- Long-Term Data : Share degradation kinetics datasets (e.g., conductivity vs. time) in discipline-specific repositories like Zenodo or Materials Cloud .
Q. Advanced: How can computational modeling resolve contradictions in GeO’s thermal expansion coefficients reported across studies?
Methodological Answer:
Thermal expansion variability often stems from anisotropic crystal structures or measurement artifacts. A systematic workflow includes:
- DFT Phonon Calculations : Simulate thermal expansion coefficients (TECs) using quasi-harmonic approximation (QHA) in software like Quantum ESPRESSO. Compare with experimental XRD data collected at multiple temperatures .
- Error Analysis : Quantify instrument-induced errors (e.g., dilatometer calibration drift) and publish raw thermal expansion curves .
- Community Benchmarking : Contribute data to open platforms like the Materials Project, enabling meta-analyses of TEC trends .
Q. Basic: What are the best practices for managing and sharing GeO research data to comply with funding agency requirements?
Methodological Answer:
Adhere to FAIR principles (Findable, Accessible, Interoperable, Reusable):
- Metadata Standards : Use the Crystallographic Information Framework (CIF) for structural data and include experimental conditions (e.g., synthesis temperature, pressure) .
- Repositories : Upload datasets to discipline-specific platforms (e.g., ICSD for crystal structures, NOMAD for computational outputs) with persistent identifiers (DOIs) .
- Data Management Plans (DMPs) : Align with DFG/NSF checklists, specifying retention periods (minimum 10 years) and access protocols .
Q. Advanced: How to design experiments distinguishing GeO’s intrinsic semiconductor properties from surface oxidation artifacts?
Methodological Answer:
Surface oxidation (GeO → GeO₂) can mask intrinsic properties. Experimental design tips:
- In Situ Characterization : Use synchrotron-based XPS or Raman spectroscopy under ultra-high vacuum (UHV) to probe bulk vs. surface states .
- Passivation Methods : Etch surfaces with dilute HF (1–5%) and immediately coat with inert layers (e.g., graphene) for electrical measurements .
- Control Experiments : Compare samples stored in argon vs. ambient conditions, reporting time-resolved conductivity/PL spectra .
Q. Advanced: What statistical methods are robust for analyzing conflicting catalytic activity data of GeO in CO oxidation reactions?
Methodological Answer:
Address variability via:
- Multivariate Regression : Correlate activity with surface area, defect density (from TEM), and pretreatment conditions (e.g., reduction temperature) .
- Error-Weighted Averaging : Apply Bayesian inference to reconcile disparate activity measurements, prioritizing datasets with detailed uncertainty quantification .
- Reproducibility Tests : Publish step-by-step catalytic testing protocols (e.g., gas flow rates, catalyst loading) in supplementary materials, inviting third-party validation .
Properties
IUPAC Name |
oxogermane | |
---|---|---|
Source | PubChem | |
URL | https://pubchem.ncbi.nlm.nih.gov | |
Description | Data deposited in or computed by PubChem | |
InChI |
InChI=1S/GeH2O/c1-2/h1H2 | |
Source | PubChem | |
URL | https://pubchem.ncbi.nlm.nih.gov | |
Description | Data deposited in or computed by PubChem | |
InChI Key |
DFIYWQBRYUCBMH-UHFFFAOYSA-N | |
Source | PubChem | |
URL | https://pubchem.ncbi.nlm.nih.gov | |
Description | Data deposited in or computed by PubChem | |
Canonical SMILES |
O=[GeH2] | |
Source | PubChem | |
URL | https://pubchem.ncbi.nlm.nih.gov | |
Description | Data deposited in or computed by PubChem | |
Molecular Formula |
GeH2O | |
Source | PubChem | |
URL | https://pubchem.ncbi.nlm.nih.gov | |
Description | Data deposited in or computed by PubChem | |
Molecular Weight |
90.65 g/mol | |
Source | PubChem | |
URL | https://pubchem.ncbi.nlm.nih.gov | |
Description | Data deposited in or computed by PubChem | |
CAS No. |
20619-16-3 | |
Record name | Germanium oxide (GeO) | |
Source | ChemIDplus | |
URL | https://pubchem.ncbi.nlm.nih.gov/substance/?source=chemidplus&sourceid=0020619163 | |
Description | ChemIDplus is a free, web search system that provides access to the structure and nomenclature authority files used for the identification of chemical substances cited in National Library of Medicine (NLM) databases, including the TOXNET system. | |
Record name | Germanium oxide (GeO) | |
Source | EPA Chemicals under the TSCA | |
URL | https://www.epa.gov/chemicals-under-tsca | |
Description | EPA Chemicals under the Toxic Substances Control Act (TSCA) collection contains information on chemicals and their regulations under TSCA, including non-confidential content from the TSCA Chemical Substance Inventory and Chemical Data Reporting. | |
Disclaimer and Information on In-Vitro Research Products
Please be aware that all articles and product information presented on BenchChem are intended solely for informational purposes. The products available for purchase on BenchChem are specifically designed for in-vitro studies, which are conducted outside of living organisms. In-vitro studies, derived from the Latin term "in glass," involve experiments performed in controlled laboratory settings using cells or tissues. It is important to note that these products are not categorized as medicines or drugs, and they have not received approval from the FDA for the prevention, treatment, or cure of any medical condition, ailment, or disease. We must emphasize that any form of bodily introduction of these products into humans or animals is strictly prohibited by law. It is essential to adhere to these guidelines to ensure compliance with legal and ethical standards in research and experimentation.