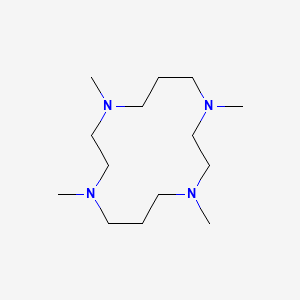
1,4,8,11-Tetramethyl-1,4,8,11-tetraazacyclotetradecane
Overview
Description
1,4,8,11-Tetramethyl-1,4,8,11-tetraazacyclotetradecane (TMC) is a macrocyclic tetramine ligand with four methyl groups substituted on its nitrogen atoms. Its rigid yet flexible structure enables the stabilization of transition metal ions in diverse coordination geometries, making it pivotal in catalysis, materials science, and bioinorganic chemistry. Key properties include:
- Structure: The 14-membered macrocycle adopts a trans-I configuration when coordinating metals, as observed in cobalt(II) complexes .
- Metal Binding: TMC stabilizes high-valent metal-oxo intermediates, such as FeIV=O and CuII–O species, critical for oxidation catalysis .
- Applications: Used in water oxidation catalysts (WOCs), molecular sieves (e.g., SSZ-79), and studies of proton-coupled electron transfer (PCET) .
Preparation Methods
Synthesis of the Parent Macrocycle: 1,4,8,11-Tetraazacyclotetradecane (Cyclam)
Cyclam serves as the foundational precursor for Tmcy. Two industrial processes dominate its synthesis, both involving multistep reactions to construct the 14-membered macrocyclic ring.
Bisacylation-Cyclization-Reduction Method
Developed by , this three-step method begins with bisacylation of 1,3-diaminopropane using chloroacetyl chloride in the presence of potassium carbonate and methylene chloride at 0–30°C . The dichlorodiamide intermediate undergoes cyclization with 1,3-diaminopropane in acetonitrile at 78–83°C for 20–30 hours, yielding dioxocyclam . Final reduction with Red-Al® in toluene at 20–35°C produces cyclam . While scalable, this route requires stringent temperature control and hazardous reagents like chlorinated solvents.
Tetratosylation-Cyclization-Detosylation Method
An alternative approach from involves tetratosylation of N,N'-bis(3-aminopropyl)ethylenediamine with p-toluenesulfonyl chloride in tetrahydrofuran (55–75°C), followed by cyclization using ethyleneglycol ditosylate and detosylation with hydrobromic acid . Though effective, this method suffers from lengthy reaction times (3–5 hours per step) and toxic byproducts .
N-Tetramethylation of Cyclam to 1,4,8,11-Tetramethyl-1,4,8,11-tetraazacyclotetradecane
N-tetramethylation introduces methyl groups to cyclam’s nitrogen atoms. Key strategies include reductive amination and direct alkylation, each with distinct advantages and limitations.
Direct Alkylation Using Methyl Halides
Direct alkylation with methyl halides (e.g., methyl iodide) in biphasic systems has been explored but faces challenges. In anhydrous DMF with excess sodium bicarbonate, competing over-alkylation leads to quaternary amine byproducts, reducing yields to 25–40% . For example, Trabaud et al. reported 60% yields for ethyl bromoacetate derivatives but noted poor reproducibility with methyl halides .
Biphasic Solvent System Approach
A novel biphasic method from employs acetonitrile and aqueous sodium hydroxide (1:1 v/v) with vigorous shaking. Although optimized for propargyl and benzyl groups, this system could theoretically adapt to methyl halides. For propargyl bromide, yields reached 90% after six hours at room temperature, suggesting potential for methyl analogues . However, methyl halides’ higher volatility and reactivity may necessitate modified conditions.
Comparative Analysis of Methylation Methods
The table below contrasts key parameters for Tmcy synthesis routes:
*Reported as efficient but unquantified; †Demonstrated for propargyl/benzyl groups.
Chemical Reactions Analysis
Oxidation Reactions
TMTAC undergoes oxidation under controlled conditions. Reaction with hydrogen peroxide (H₂O₂) in aqueous acidic media generates oxidized derivatives, primarily through N-demethylation or macrocycle ring modification . For example:
Key conditions :
-
Temperature: 25–50°C
-
Solvent: Water or ethanol/water mixtures
-
Catalysts: Transition metal ions (e.g., Cu²⁺) enhance reaction rates .
Reduction Reactions
Reductive pathways are less common but observed with sodium borohydride (NaBH₄) in non-aqueous solvents. Reduction typically targets imine-like intermediates formed during partial oxidation:
Experimental notes :
Substitution Reactions
Methyl groups on TMTAC’s nitrogen atoms participate in nucleophilic substitution under alkaline conditions. For instance, halogenation with bromine (Br₂) in NaOH yields bromomethyl derivatives:
Key parameters :
Metal Complexation Reactions
TMTAC’s primary application lies in forming stable complexes with transition metals , leveraging its tetradentate ligand structure.
Mechanistic insights :
-
Square-planar geometry dominates for Ni(II) and Cu(II) complexes .
-
Zn(II) complexes adopt tetrahedral configurations in non-aqueous solvents .
Reactions with Group 15 Elements
TMTAC reacts with AsCl₃ and SbCl₃ to form ionic adducts. For example:
Crystallographic data :
-
The arsenic complex forms a distorted octahedral anion () paired with protonated TMTAC .
-
Antimony analogues exhibit similar structural motifs but with anions .
Solvent-Dependent Reactivity
TMTAC’s coordination behavior varies significantly with solvent polarity:
Stability and Degradation Pathways
Under harsh conditions (pH < 2 or > 12), TMTAC undergoes hydrolysis or demethylation :
Degradation products include 1,4,8,11-tetraazacyclotetradecane (cyclam) and formaldehyde .
Scientific Research Applications
Coordination Chemistry
TMC serves as an excellent ligand for various metal ions due to its ability to form stable complexes. The nitrogen atoms in the macrocycle can coordinate with metals such as cobalt, iron, and nickel.
- Metal Complex Formation : TMC forms stable complexes with transition metals, which are utilized in various catalytic processes. For instance, the complexation of TMC with iron leads to the formation of non-heme iron(IV)-oxo complexes that are crucial for catalytic oxidation reactions .
Catalytic Applications
The ability of TMC to stabilize metal ions enhances its utility in catalysis:
- Oxidation Reactions : TMC-based metal complexes have been shown to catalyze oxidation reactions effectively. For example, the oxidation of TMC itself results in the formation of reactive intermediates that can facilitate further chemical transformations .
- Electrocatalysis : TMC complexes have been employed in electrocatalytic applications due to their favorable redox properties. They can enhance the efficiency of electrochemical reactions by providing a stable environment for electron transfer processes .
Biological Applications
TMC's properties extend into biological systems where it can interact with biomolecules:
- Metal Ion Chelation : In biological contexts, TMC acts as a chelator for essential metal ions such as copper and iron. This property is significant for maintaining metal ion homeostasis in living organisms and has implications in drug design where metal ions play a critical role .
- Anticancer Research : The interaction of TMC-based complexes with cellular redox systems has garnered attention in cancer research. These complexes can modulate redox signaling pathways and potentially serve as anticancer agents by disturbing the redox balance within cancer cells .
Material Science
In material science, TMC is used to develop new materials with specific properties:
- Nanomaterials : TMC derivatives are explored for synthesizing nanostructured materials that exhibit unique electronic and optical properties. These materials have potential applications in sensors and electronic devices due to their enhanced conductivity and stability .
Case Study 1: Iron Complexes
Research has demonstrated that TMC can stabilize various iron oxidation states, leading to the formation of complexes that exhibit unique catalytic activities. For instance, the non-heme iron(IV)-oxo complex derived from TMC has shown promise in oxidizing organic substrates efficiently .
Case Study 2: Photovoltaic Applications
TMC-based cobalt complexes have been investigated as dopants in solar cells. These complexes enhance the performance of photovoltaic devices by improving charge transport properties and overall efficiency .
Mechanism of Action
The mechanism of action of 1,4,8,11-tetramethyl-1,4,8,11-tetraazacyclotetradecane involves its ability to chelate metal ions. The nitrogen atoms in the macrocyclic ring coordinate with metal ions, forming stable complexes. This chelation can alter the properties of the metal ions, making them useful in various chemical and biological processes .
Comparison with Similar Compounds
Structural and Functional Analogues
1,4,8,11-Tetraazacyclotetradecane-1,4,8,11-tetraacetic acid (TETA)
- Structural Differences : TETA replaces TMC’s methyl groups with acetic acid moieties, enhancing water solubility and altering metal-binding affinity .
- Metal Coordination : Preferentially binds lanthanides (e.g., Gd³⁺, Eu³⁺) and radiometals (e.g., Cu-64, Ga-68) for medical imaging .
- Catalytic Role : Less effective than TMC in stabilizing high-valent metal-oxo species due to weaker ligand field strength .
1,4,8,11-Tetraazacyclotetradecane-1,4,8,11-tetrapropionic acid (TETPA)
- Modifications : Propionic acid substituents increase steric bulk and hydrophilicity compared to TMC.
- Applications : Primarily used in radiopharmaceuticals (e.g., PSMA-targeted agents) rather than catalysis .
12-TMC and 15-TMC
- Ring Size : 12-TMC (12-membered) and 15-TMC (15-membered) exhibit distinct cavity sizes, affecting metal ion selectivity. For example, 12-TMC favors smaller ions like Fe²⁺, while 14-TMC (TMC) accommodates larger ions like Cu²⁺ .
- Catalytic Performance: 14-TMC-based CuII complexes show higher turnover frequencies (TOF = 30 s⁻¹) in water oxidation compared to smaller analogues due to optimized ligand flexibility and counter-ion interactions .
Catalytic Performance in Water Oxidation
Metal-Oxo Complex Stability
- FeIV=O Stretching Frequency : TMC-based FeIV=O complexes exhibit Fe–O vibrations at 848 cm⁻¹ , shifting to 812 cm⁻¹ with ¹⁸O isotope substitution. This contrasts with porphyrin-based FeIV=O systems (~820 cm⁻¹), where stronger π-donation lowers vibrational energy .
- Redox-Inactive Metal Binding : TMC’s FeIV=O moiety interacts with Sc³⁺, inducing structural distortion and altering electron transfer pathways—a feature absent in simpler ligands like salen .
Biological Activity
1,4,8,11-Tetramethyl-1,4,8,11-tetraazacyclotetradecane (TMC) is a macrocyclic compound known for its chelating properties and potential biological applications. This compound has garnered attention in various fields, including medicinal chemistry and catalysis. This article explores the biological activity of TMC, focusing on its mechanisms of action, interactions with biological systems, and relevant case studies.
- Molecular Formula : C₁₄H₃₂N₄
- Molecular Weight : 256.43 g/mol
- CAS Number : 41203-22-9
TMC is characterized by a tetraazamacrocyclic structure that allows it to form stable complexes with metal ions. Its structural features contribute significantly to its biological activity.
Mechanisms of Biological Activity
TMC exhibits various biological activities primarily through its role as a ligand in coordination chemistry. Its ability to chelate metal ions enhances its reactivity and interaction with biological molecules.
Chelation and Metal Ion Interaction
TMC is known to form complexes with transition metals such as copper(II). These complexes can influence enzymatic reactions and cellular processes. For instance, studies have shown that TMC can stabilize copper ions in biological systems, which is crucial for enzymatic functions involving copper-dependent enzymes .
Antioxidant Activity
Research indicates that TMC may possess antioxidant properties. The chelation of metal ions can prevent oxidative stress by reducing the availability of free radicals. This mechanism is particularly relevant in neuroprotection and anti-inflammatory responses .
Study 1: Copper Complexes and Electrocatalysis
A study investigated the electrocatalytic properties of copper(II) complexes formed with TMC. The results demonstrated that these complexes could facilitate oxygen evolution reactions, highlighting their potential application in energy conversion technologies. The kinetic isotope effect (KIE) observed was indicative of a proton-independent mechanism during the rate-limiting step of the reaction .
Study 2: Antioxidant Mechanism in Neuroprotection
Another research focused on the neuroprotective effects of TMC in models of oxidative stress-induced neuronal damage. The findings suggested that TMC's ability to chelate metal ions reduced neuronal cell death by mitigating oxidative damage. This study underscores TMC's potential as a therapeutic agent in neurodegenerative diseases .
Data Table: Summary of Biological Activities
Q & A
Basic Research Questions
Q. What are the critical safety protocols for handling 1,4,8,11-tetraazacyclotetradecane in laboratory settings?
- Methodological Guidance :
-
Hazard Identification : The compound is classified under GHS as causing skin irritation (H315), serious eye irritation (H319), and respiratory tract irritation (H335) .
-
First Aid Measures : In case of inhalation, move to fresh air and provide artificial respiration if necessary. For skin/eye contact, rinse immediately with water for 15 minutes and seek medical attention .
-
Storage : Store at 0–6°C in tightly sealed containers to prevent degradation .
Hazard Category GHS Code Precautionary Measures Skin Irritation H315 Use nitrile gloves and lab coats. Eye Irritation H319 Wear safety goggles. Respiratory Irritant H335 Use fume hoods and N95 respirators.
Q. How can researchers synthesize and purify 1,4,8,11-tetraazacyclotetradecane derivatives for functionalization studies?
- Methodological Guidance :
- Synthetic Routes : Derivatives like 1,1'-[1,4-phenylenebis(methylene)]bis-tetraazacyclotetradecane (CAS 155148-31-5) are synthesized via alkylation or benzylation of the parent macrocycle. Optimize reaction conditions (e.g., solvent polarity, temperature) to minimize side products .
- Purification : Use column chromatography with polar stationary phases (e.g., silica gel) and methanol/ethyl acetate gradients. Confirm purity via HPLC or NMR .
Q. What spectroscopic techniques are most effective for characterizing the structural conformation of 1,4,8,11-tetraazacyclotetradecane?
- Methodological Guidance :
- NMR : Use - and -NMR to identify proton environments and confirm macrocyclic symmetry. For example, methyl groups at positions 1,4,8,11 show distinct splitting patterns .
- X-ray Crystallography : Resolve crystal structures to analyze bond angles and coordination geometry (e.g., tetraazoniacyclotetradecane sulfate structures in Acta Crystallographica) .
Advanced Research Questions
Q. How can factorial design optimize reaction parameters for synthesizing 1,4,8,11-tetraazacyclotetradecane-metal complexes?
- Methodological Guidance :
-
Variables : Test factors like temperature (25–80°C), solvent (water vs. acetonitrile), and ligand-to-metal ratio (1:1 to 1:4). Use a 2 factorial design to identify significant interactions .
-
Response Metrics : Monitor complex stability via UV-Vis spectroscopy (e.g., absorbance shifts at λ~250 nm for Cu complexes) .
Factor Range Tested Optimal Condition Temperature 25–80°C 60°C Solvent Polarity Water, Acetonitrile Acetonitrile Ligand:Metal Ratio 1:1 to 1:4 1:2
Q. What computational methods predict the coordination behavior of 1,4,8,11-tetraazacyclotetradecane with transition metals?
- Methodological Guidance :
- DFT Calculations : Use Gaussian or ORCA software to model metal-ligand bond lengths and electronic properties. Compare with experimental data (e.g., Cu complexation in Bulletin of the Academy of Sciences) .
- Molecular Dynamics : Simulate solvation effects in aqueous vs. non-polar environments to assess thermodynamic stability .
Q. How do researchers resolve contradictions in thermodynamic stability data for 1,4,8,11-tetraazacyclotetradecane complexes?
- Methodological Guidance :
- Data Reconciliation : Compare stability constants (log K) across studies. For example, discrepancies in Cu complex stability may arise from pH variations or ionic strength differences .
- Controlled Replication : Standardize buffer systems (e.g., 0.1 M KCl for ionic strength control) and use potentiometric titrations with a glass electrode .
Q. What strategies enhance the selectivity of 1,4,8,11-tetraazacyclotetradecane as a chelating agent for rare-earth elements?
- Methodological Guidance :
- Side-Chain Functionalization : Introduce phosphonate or carboxylate groups (e.g., dihydroxyphosphorylmethyl derivatives) to improve metal affinity .
- pH-Dependent Studies : Adjust solution pH to leverage protonation states of the macrocycle. For example, La binding is optimal at pH 5–6 .
Q. Methodological Resources
- Structural Databases : PubChem (CID 295-37-4) for molecular descriptors and toxicity profiles .
- Safety Compliance : Follow OSHA HCS guidelines for hazard communication .
Properties
IUPAC Name |
1,4,8,11-tetramethyl-1,4,8,11-tetrazacyclotetradecane | |
---|---|---|
Source | PubChem | |
URL | https://pubchem.ncbi.nlm.nih.gov | |
Description | Data deposited in or computed by PubChem | |
InChI |
InChI=1S/C14H32N4/c1-15-7-5-8-17(3)13-14-18(4)10-6-9-16(2)12-11-15/h5-14H2,1-4H3 | |
Source | PubChem | |
URL | https://pubchem.ncbi.nlm.nih.gov | |
Description | Data deposited in or computed by PubChem | |
InChI Key |
HRFJEOWVAGSJNW-UHFFFAOYSA-N | |
Source | PubChem | |
URL | https://pubchem.ncbi.nlm.nih.gov | |
Description | Data deposited in or computed by PubChem | |
Canonical SMILES |
CN1CCCN(CCN(CCCN(CC1)C)C)C | |
Source | PubChem | |
URL | https://pubchem.ncbi.nlm.nih.gov | |
Description | Data deposited in or computed by PubChem | |
Molecular Formula |
C14H32N4 | |
Source | PubChem | |
URL | https://pubchem.ncbi.nlm.nih.gov | |
Description | Data deposited in or computed by PubChem | |
DSSTOX Substance ID |
DTXSID10194126 | |
Record name | 1,4,8,11-Tetraazacyclotetradecane, 1,4,8,11-tetramethyl- | |
Source | EPA DSSTox | |
URL | https://comptox.epa.gov/dashboard/DTXSID10194126 | |
Description | DSSTox provides a high quality public chemistry resource for supporting improved predictive toxicology. | |
Molecular Weight |
256.43 g/mol | |
Source | PubChem | |
URL | https://pubchem.ncbi.nlm.nih.gov | |
Description | Data deposited in or computed by PubChem | |
CAS No. |
41203-22-9 | |
Record name | 1,4,8,11-Tetraazacyclotetradecane, 1,4,8,11-tetramethyl- | |
Source | ChemIDplus | |
URL | https://pubchem.ncbi.nlm.nih.gov/substance/?source=chemidplus&sourceid=0041203229 | |
Description | ChemIDplus is a free, web search system that provides access to the structure and nomenclature authority files used for the identification of chemical substances cited in National Library of Medicine (NLM) databases, including the TOXNET system. | |
Record name | 1,4,8,11-Tetraazacyclotetradecane, 1,4,8,11-tetramethyl- | |
Source | EPA DSSTox | |
URL | https://comptox.epa.gov/dashboard/DTXSID10194126 | |
Description | DSSTox provides a high quality public chemistry resource for supporting improved predictive toxicology. | |
Record name | 1,4,8,11-Tetramethyl-1,4,8,11-tetraazacyclotetradecane | |
Source | European Chemicals Agency (ECHA) | |
URL | https://echa.europa.eu/information-on-chemicals | |
Description | The European Chemicals Agency (ECHA) is an agency of the European Union which is the driving force among regulatory authorities in implementing the EU's groundbreaking chemicals legislation for the benefit of human health and the environment as well as for innovation and competitiveness. | |
Explanation | Use of the information, documents and data from the ECHA website is subject to the terms and conditions of this Legal Notice, and subject to other binding limitations provided for under applicable law, the information, documents and data made available on the ECHA website may be reproduced, distributed and/or used, totally or in part, for non-commercial purposes provided that ECHA is acknowledged as the source: "Source: European Chemicals Agency, http://echa.europa.eu/". Such acknowledgement must be included in each copy of the material. ECHA permits and encourages organisations and individuals to create links to the ECHA website under the following cumulative conditions: Links can only be made to webpages that provide a link to the Legal Notice page. | |
Retrosynthesis Analysis
AI-Powered Synthesis Planning: Our tool employs the Template_relevance Pistachio, Template_relevance Bkms_metabolic, Template_relevance Pistachio_ringbreaker, Template_relevance Reaxys, Template_relevance Reaxys_biocatalysis model, leveraging a vast database of chemical reactions to predict feasible synthetic routes.
One-Step Synthesis Focus: Specifically designed for one-step synthesis, it provides concise and direct routes for your target compounds, streamlining the synthesis process.
Accurate Predictions: Utilizing the extensive PISTACHIO, BKMS_METABOLIC, PISTACHIO_RINGBREAKER, REAXYS, REAXYS_BIOCATALYSIS database, our tool offers high-accuracy predictions, reflecting the latest in chemical research and data.
Strategy Settings
Precursor scoring | Relevance Heuristic |
---|---|
Min. plausibility | 0.01 |
Model | Template_relevance |
Template Set | Pistachio/Bkms_metabolic/Pistachio_ringbreaker/Reaxys/Reaxys_biocatalysis |
Top-N result to add to graph | 6 |
Feasible Synthetic Routes
Disclaimer and Information on In-Vitro Research Products
Please be aware that all articles and product information presented on BenchChem are intended solely for informational purposes. The products available for purchase on BenchChem are specifically designed for in-vitro studies, which are conducted outside of living organisms. In-vitro studies, derived from the Latin term "in glass," involve experiments performed in controlled laboratory settings using cells or tissues. It is important to note that these products are not categorized as medicines or drugs, and they have not received approval from the FDA for the prevention, treatment, or cure of any medical condition, ailment, or disease. We must emphasize that any form of bodily introduction of these products into humans or animals is strictly prohibited by law. It is essential to adhere to these guidelines to ensure compliance with legal and ethical standards in research and experimentation.