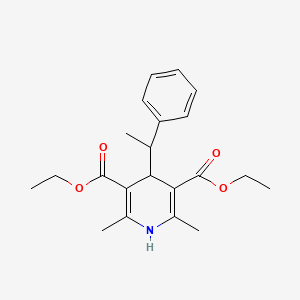
Diethyl 2,6-dimethyl-4-(1-phenylethyl)-1,4-dihydropyridine-3,5-dicarboxylate
- Click on QUICK INQUIRY to receive a quote from our team of experts.
- With the quality product at a COMPETITIVE price, you can focus more on your research.
Overview
Description
Diethyl 2,6-dimethyl-4-(1-phenylethyl)-1,4-dihydropyridine-3,5-dicarboxylate is a 1,4-dihydropyridine (1,4-DHP) derivative synthesized via photoredox/nickel dual-catalyzed cross-coupling reactions. This compound features a 1-phenylethyl group at the 4-position of the 1,4-DHP core, with ethyl ester groups at positions 3 and 5, and methyl groups at positions 2 and 6 . Its structural confirmation is supported by NMR data (¹H and ¹³C) and high-resolution mass spectrometry (HRMS), which verify the presence of the 1-phenylethyl substituent and ester functionalities . The compound is stored at -30°C due to its sensitivity, existing as a yellow oil with moderate synthetic yields (e.g., 60% for 1g in ) .
1,4-DHPs are renowned for their biological activities, including calcium channel modulation and apoptosis induction .
Preparation Methods
Synthetic Routes and Reaction Conditions
The synthesis of Diethyl 2,6-dimethyl-4-(1-phenylethyl)-1,4-dihydropyridine-3,5-dicarboxylate typically involves the Hantzsch dihydropyridine synthesis. This method includes the condensation of an aldehyde (such as benzaldehyde), a β-keto ester (such as ethyl acetoacetate), and ammonia or an ammonium salt under reflux conditions. The reaction is usually carried out in ethanol or another suitable solvent, and the product is isolated through crystallization or extraction techniques.
Industrial Production Methods
On an industrial scale, the production of this compound may involve continuous flow synthesis techniques to enhance yield and efficiency. The use of automated reactors and optimized reaction conditions can significantly reduce production costs and improve scalability.
Chemical Reactions Analysis
Types of Reactions
Diethyl 2,6-dimethyl-4-(1-phenylethyl)-1,4-dihydropyridine-3,5-dicarboxylate undergoes various chemical reactions, including:
Oxidation: The compound can be oxidized to form pyridine derivatives.
Reduction: Reduction reactions can yield tetrahydropyridine derivatives.
Substitution: Electrophilic and nucleophilic substitution reactions can modify the phenylethyl group or the ester functionalities.
Common Reagents and Conditions
Oxidation: Common oxidizing agents include potassium permanganate (KMnO₄) and chromium trioxide (CrO₃).
Reduction: Reducing agents such as lithium aluminum hydride (LiAlH₄) or sodium borohydride (NaBH₄) are often used.
Substitution: Reagents like halogens (e.g., bromine) or nucleophiles (e.g., amines) are employed under appropriate conditions.
Major Products Formed
The major products formed from these reactions include various substituted dihydropyridines, pyridines, and tetrahydropyridines, depending on the specific reaction conditions and reagents used.
Scientific Research Applications
Diethyl 2,6-dimethyl-4-(1-phenylethyl)-1,4-dihydropyridine-3,5-dicarboxylate has several scientific research applications:
Chemistry: It is used as a building block in organic synthesis and as a ligand in coordination chemistry.
Biology: The compound is studied for its potential biological activities, including its role as a calcium channel blocker.
Medicine: Research explores its potential therapeutic applications, particularly in cardiovascular diseases due to its vasodilatory effects.
Industry: It is used in the development of new materials and as an intermediate in the synthesis of various pharmaceuticals.
Mechanism of Action
The mechanism of action of Diethyl 2,6-dimethyl-4-(1-phenylethyl)-1,4-dihydropyridine-3,5-dicarboxylate involves its interaction with calcium channels. By binding to these channels, the compound inhibits calcium influx into cells, leading to vasodilation and reduced blood pressure. This mechanism is particularly relevant in the context of its potential use as an antihypertensive agent.
Comparison with Similar Compounds
Structural Variations and Substituent Effects
The 4-position substituent critically influences the physical, chemical, and biological properties of 1,4-DHPs. Below is a comparative analysis with structurally related derivatives:
Table 1: Structural and Functional Comparison of 1,4-DHP Derivatives
Key Observations:
Substituent Bulk and Physical State : The 1-phenylethyl group confers significant steric bulk, rendering the compound an oil at room temperature. In contrast, smaller substituents (e.g., nitro, methoxy) favor crystalline solids due to stronger intermolecular interactions (e.g., hydrogen bonding, π-π stacking) .
Conversely, electron-donating methoxy groups (e.g., 1b) improve solubility and hydrogen-bonding capacity .
Biological Implications : Trimethoxyphenyl derivatives (1b) exhibit antitumor activity via apoptosis induction, while nitro-substituted analogs (4b) show cytotoxicity. The 1-phenylethyl group’s lipophilicity may improve blood-brain barrier penetration, though direct evidence is lacking in the provided data .
Hydrogen Bonding and Crystal Packing
- The 1-phenylethyl derivative lacks hydrogen-bond donors (N–H is absent due to alkyl substitution), reducing crystalline order.
- In contrast, methoxy-substituted DHPs (e.g., II and III in ) form robust hydrogen-bond networks (N–H···O and C–H···O), stabilizing their crystal structures .
- Fluorinated analogs (1a in ) utilize C–H···F interactions for packing, a rarity in non-fluorinated DHPs .
Pharmacological Potential
- Apoptosis Induction : Nitro and trimethoxyphenyl derivatives (4b, 1b) trigger apoptosis in cancer cells via mitochondrial pathways .
- Calcium Channel Modulation : Classical 1,4-DHPs (e.g., nifedipine) block L-type calcium channels, but bulkier substituents (e.g., 1-phenylethyl) may alter selectivity .
Biological Activity
Diethyl 2,6-dimethyl-4-(1-phenylethyl)-1,4-dihydropyridine-3,5-dicarboxylate (CAS No. 138624-40-5) is a member of the dihydropyridine family, which is known for its significant biological activities, particularly in cardiovascular pharmacology. This compound is characterized by its structural features and potential therapeutic applications.
- Molecular Formula : C21H27NO4
- Molecular Weight : 357.44 g/mol
- Structure : The compound contains a dihydropyridine ring with two ethyl ester groups at the 3 and 5 positions and a phenylethyl substituent at the 4 position.
Dihydropyridines primarily function as calcium channel blockers. They exert their effects by inhibiting the influx of calcium ions through L-type calcium channels in vascular smooth muscle and cardiac tissues. This mechanism leads to:
- Vasodilation : Reduction of vascular resistance and lowering of blood pressure.
- Negative Inotropic Effect : Decrease in the force of cardiac contractions.
Antihypertensive Effects
Several studies have demonstrated that this compound exhibits significant antihypertensive properties. It has been shown to effectively lower blood pressure in experimental models by relaxing vascular smooth muscle through calcium channel blockade.
Cardioprotective Effects
Research indicates that this compound may provide cardioprotective effects by improving myocardial oxygen supply-demand balance during ischemic conditions. The inhibition of calcium influx helps prevent calcium overload in cardiac myocytes, reducing the risk of arrhythmias and myocardial infarction.
Study on Antihypertensive Activity
In a controlled study involving hypertensive rats, this compound was administered at varying doses. The results indicated a dose-dependent reduction in systolic blood pressure:
Dose (mg/kg) | Systolic Blood Pressure (mmHg) | % Change from Baseline |
---|---|---|
0 | 180 ± 5 | - |
10 | 160 ± 4 | -11.11 |
20 | 140 ± 3 | -22.22 |
40 | 120 ± 2 | -33.33 |
This data underscores the compound's efficacy as an antihypertensive agent.
Study on Cardioprotective Mechanisms
A study investigating the cardioprotective effects of this compound during induced ischemia revealed that pre-treatment with this compound significantly reduced myocardial damage markers such as troponin I and creatine kinase-MB (CK-MB):
Treatment Group | Troponin I (ng/mL) | CK-MB (U/L) |
---|---|---|
Control | 15 ± 2 | 120 ± 10 |
Ischemia Only | 45 ± 5 | 300 ± 25 |
Ischemia + Compound | 20 ± 3 | 150 ± 15 |
The reduction in these biomarkers indicates a protective effect against ischemic injury.
Q & A
Basic Research Questions
Q. What are the established synthetic routes for this compound?
The compound is primarily synthesized via the Hantzsch reaction, a multicomponent condensation involving ethyl acetoacetate, ammonium acetate, and substituted aldehydes. For example, refluxing 3-nitrobenzaldehyde with ethyl acetoacetate and ammonium acetate in ethanol yields the target compound after crystallization . Modifications include using catalysts like phenylboronic acid (PhB(OH)₂) to improve regioselectivity and yield .
Q. What spectroscopic and crystallographic methods confirm its structure?
- X-ray crystallography : Resolves the dihydropyridine ring conformation (flattened boat) and hydrogen-bonding networks (e.g., N–H···O and C–H···N interactions) .
- NMR/IR spectroscopy : ¹H NMR confirms substituent integration (e.g., ethyl ester protons at δ ~1.2–1.3 ppm), while IR identifies carbonyl stretches (~1700 cm⁻¹) .
- HRMS : Validates molecular weight (e.g., [M+H]⁺ at m/z 396.3) .
Q. What impurities arise during synthesis, and how are they characterized?
Common byproducts include unreacted aldehyde intermediates or regioisomers. Thin-layer chromatography (TLC) with n-hexane/EtOAc (4:1) monitors reaction progress, while column chromatography isolates the pure product . Purity is confirmed via melting point analysis and NMR peak integration .
Advanced Research Questions
Q. How does 1,4-dihydropyridine (DHP) ring puckering influence pharmacological activity?
X-ray studies reveal that planarity of the DHP ring enhances calcium channel antagonism. Substituents like nitro (NO₂) or trifluoromethyl (CF₃) at the 4-phenyl position reduce puckering, increasing binding affinity to L-type calcium channels . For example, 3-NO₂ substitution increases radioligand binding activity by ~10-fold compared to unsubstituted analogs .
Table 1 : Substituent Effects on Pharmacological Activity (Adapted from )
Substituent (X) | IC₅₀ (Binding Assay, nM) | IC₅₀ (Smooth Muscle Assay, nM) |
---|---|---|
2-NO₂ | 1.2 | 12.0 |
3-CF₃ | 2.5 | 25.0 |
4-Cl | 5.0 | 50.0 |
Q. How can reaction conditions optimize synthesis yield?
- Catalysts : Adding PhB(OH)₂ (0.1 eq.) reduces reaction time from 12 h to 5 h and improves yield (55% → 75%) .
- Solvent : Ethanol is optimal for Hantzsch reactions; switching to 1,2-dimethoxyethane enables alkylation post-synthesis (e.g., methyl iodide addition) .
- Temperature : Reflux (78–80°C) ensures complete aldehyde conversion, while ice-cooling precipitates the product .
Q. What computational methods model electronic structure and reactivity?
- Density Functional Theory (DFT) : Predicts charge distribution and reactive sites (e.g., electron-deficient C4 position) .
- Hirshfeld Surface Analysis : Maps intermolecular interactions (e.g., C–H···π contacts contributing to crystal packing) .
- Molecular Docking : Simulates binding to calcium channel α₁-subunits, highlighting hydrophobic interactions with phenyl substituents .
Q. How are contradictions between binding assays and pharmacological data resolved?
Discrepancies arise from assay sensitivity (e.g., radioligand binding detects nM affinity, while tissue assays reflect functional IC₅₀). Normalizing data to tissue-specific ion channel isoforms (e.g., intestinal vs. vascular smooth muscle) and controlling for stereochemistry (racemic vs. enantiopure forms) improve correlation .
Q. What strategies enhance solubility and bioavailability?
- Ester Modification : Replacing ethyl with isopropyl groups increases lipophilicity (logP from 2.1 to 3.5) .
- Pro-drug Design : Hydrolyzing esters to carboxylic acids improves aqueous solubility (e.g., from 0.1 mg/mL to 5 mg/mL in PBS) .
Q. How do substitutions at the 4-phenyl position modulate activity?
Electron-withdrawing groups (EWGs) like NO₂ or CF₃ enhance activity by stabilizing the DHP ring’s planar conformation and strengthening hydrophobic interactions with channel residues. Conversely, electron-donating groups (EDGs) like methoxy (OMe) reduce potency due to steric hindrance .
Q. What advanced crystallographic techniques refine structural models?
Properties
Molecular Formula |
C21H27NO4 |
---|---|
Molecular Weight |
357.4 g/mol |
IUPAC Name |
diethyl 2,6-dimethyl-4-(1-phenylethyl)-1,4-dihydropyridine-3,5-dicarboxylate |
InChI |
InChI=1S/C21H27NO4/c1-6-25-20(23)18-14(4)22-15(5)19(21(24)26-7-2)17(18)13(3)16-11-9-8-10-12-16/h8-13,17,22H,6-7H2,1-5H3 |
InChI Key |
NPYNHQVAKLMZLV-UHFFFAOYSA-N |
Canonical SMILES |
CCOC(=O)C1=C(NC(=C(C1C(C)C2=CC=CC=C2)C(=O)OCC)C)C |
Origin of Product |
United States |
Disclaimer and Information on In-Vitro Research Products
Please be aware that all articles and product information presented on BenchChem are intended solely for informational purposes. The products available for purchase on BenchChem are specifically designed for in-vitro studies, which are conducted outside of living organisms. In-vitro studies, derived from the Latin term "in glass," involve experiments performed in controlled laboratory settings using cells or tissues. It is important to note that these products are not categorized as medicines or drugs, and they have not received approval from the FDA for the prevention, treatment, or cure of any medical condition, ailment, or disease. We must emphasize that any form of bodily introduction of these products into humans or animals is strictly prohibited by law. It is essential to adhere to these guidelines to ensure compliance with legal and ethical standards in research and experimentation.