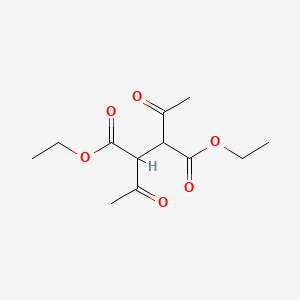
Diethyl 2,3-diacetylsuccinate
Overview
Description
Diethyl 2,3-diacetylsuccinate is an organic compound with the molecular formula C12H18O6 It is a diester derivative of succinic acid, characterized by the presence of two acetyl groups at the 2 and 3 positions
Mechanism of Action
Target of Action
Diethyl 2,3-diacetylsuccinate is primarily used as a component in titanium-magnesium catalysts for propylene polymerization . These catalysts are essential for producing stereoregular isotactic polypropylene, a type of plastic with a broad range of applications .
Mode of Action
It is known to act as astereoregulating component in titanium-magnesium catalysts . This means it helps control the spatial arrangement of atoms in the resulting polypropylene, ensuring a high degree of stereoregularity .
Biochemical Pathways
This compound is synthesized through a procedure involving the condensation of a succinic acid diester with two isobutyraldehyde molecules, followed by esterification and hydrogenation . The resulting compound is then used in the production of polypropylene via a catalytic process .
Result of Action
The use of this compound in titanium-magnesium catalysts results in the synthesis of polypropylene with a broad molecular-mass distribution . The catalysts prepared using >95% pure this compound have the best characteristics and allow preparation of polypropylene with high isotacticity index in a high yield .
Action Environment
Environmental factors can influence the action, efficacy, and stability of this compound. It’s important to note that safety precautions should be taken when handling this compound, including avoiding dust formation and ensuring adequate ventilation .
Biochemical Analysis
Biochemical Properties
Diethyl 2,3-diacetylsuccinate plays a significant role in biochemical reactions, particularly in the context of metabolic pathways involving succinate. It interacts with enzymes such as succinate dehydrogenase, which is a key component of the tricarboxylic acid cycle (TCA cycle). This interaction is crucial as succinate dehydrogenase catalyzes the oxidation of succinate to fumarate, a critical step in cellular respiration . Additionally, this compound may interact with other proteins and biomolecules involved in oxidative phosphorylation, influencing the overall energy production in cells.
Cellular Effects
This compound has been shown to affect various types of cells and cellular processes. In microglial cells, for instance, it modulates polarization and activation by reducing mitochondrial fission and cellular reactive oxygen species (ROS) production . This compound influences cell signaling pathways, particularly those related to inflammation and oxidative stress. By preventing the conversion of microglia into the pro-inflammatory M1 phenotype, this compound plays a protective role in cellular function . It also impacts gene expression and cellular metabolism, contributing to the regulation of inflammatory responses.
Molecular Mechanism
The molecular mechanism of this compound involves its interaction with specific biomolecules and enzymes. At the molecular level, this compound binds to succinate dehydrogenase, inhibiting its activity and thereby affecting the TCA cycle . This inhibition leads to a decrease in the production of fumarate and a subsequent reduction in the overall energy output of the cell. Additionally, this compound influences gene expression by modulating transcription factors and signaling pathways associated with oxidative stress and inflammation . These interactions highlight the compound’s role in regulating cellular metabolism and maintaining cellular homeostasis.
Temporal Effects in Laboratory Settings
In laboratory settings, the effects of this compound have been observed to change over time. The stability and degradation of this compound are critical factors influencing its long-term effects on cellular function. Studies have shown that this compound remains stable under controlled conditions, but its degradation products can have varying impacts on cells . Long-term exposure to this compound in in vitro and in vivo studies has demonstrated its potential to modulate cellular processes, including mitochondrial function and ROS production . These temporal effects are essential for understanding the compound’s overall impact on cellular health.
Dosage Effects in Animal Models
The effects of this compound vary with different dosages in animal models. At lower doses, the compound has been shown to exert protective effects on cellular function by reducing oxidative stress and inflammation . At higher doses, this compound can have toxic or adverse effects, including disruption of cellular metabolism and induction of apoptosis . These dosage-dependent effects highlight the importance of determining the optimal concentration for therapeutic applications while minimizing potential toxicity.
Metabolic Pathways
This compound is involved in several metabolic pathways, primarily those related to the TCA cycle. It interacts with enzymes such as succinate dehydrogenase and fumarase, influencing the conversion of succinate to fumarate and subsequently to malate . This compound also affects the levels of various metabolites, including succinate, fumarate, and malate, thereby modulating metabolic flux within the cell . The involvement of this compound in these pathways underscores its role in cellular energy production and metabolic regulation.
Transport and Distribution
The transport and distribution of this compound within cells and tissues are mediated by specific transporters and binding proteins. This compound is transported across cellular membranes via passive diffusion and facilitated transport mechanisms . Once inside the cell, this compound can accumulate in specific compartments, such as the mitochondria, where it exerts its biochemical effects . The distribution of this compound within tissues is influenced by factors such as tissue perfusion and cellular uptake mechanisms.
Subcellular Localization
This compound exhibits specific subcellular localization, primarily within the mitochondria. This localization is facilitated by targeting signals and post-translational modifications that direct the compound to the mitochondrial matrix . Within the mitochondria, this compound interacts with key enzymes involved in the TCA cycle, influencing mitochondrial function and energy production . The subcellular localization of this compound is crucial for its activity and function, as it ensures the precise modulation of metabolic pathways and cellular processes.
Preparation Methods
Synthetic Routes and Reaction Conditions: Diethyl 2,3-diacetylsuccinate can be synthesized through several methods. One common approach involves the reaction of ethyl acetoacetate with N-bromosuccinimide and potassium tert-butylate in tetrahydrofuran at room temperature . Another method includes the condensation of a succinic acid diester with two isobutyraldehyde molecules, followed by esterification and hydrogenation of the resulting dienes .
Industrial Production Methods: Industrial production of this compound typically follows similar synthetic routes but on a larger scale. The choice of method depends on factors such as yield, purity, and cost-effectiveness. Optimization of reaction conditions, such as temperature, solvent, and catalysts, is crucial for efficient production.
Chemical Reactions Analysis
Types of Reactions: Diethyl 2,3-diacetylsuccinate undergoes various chemical reactions, including:
Oxidation: This compound can be oxidized to form corresponding carboxylic acids.
Reduction: Reduction reactions can convert the acetyl groups to alcohols.
Substitution: The acetyl groups can be substituted with other functional groups under appropriate conditions.
Common Reagents and Conditions:
Oxidation: Common oxidizing agents include potassium permanganate and chromium trioxide.
Reduction: Sodium borohydride and lithium aluminum hydride are typical reducing agents.
Substitution: Reagents such as alkyl halides and nucleophiles are used for substitution reactions.
Major Products: The major products formed from these reactions depend on the specific reagents and conditions used. For example, oxidation typically yields carboxylic acids, while reduction produces alcohols.
Scientific Research Applications
Diethyl 2,3-diacetylsuccinate has various applications in scientific research:
Chemistry: It is used as an intermediate in organic synthesis, particularly in the preparation of complex molecules.
Biology: The compound serves as a tool for studying enzyme-catalyzed reactions and metabolic pathways.
Industry: this compound is used in the production of polymers and other materials with specific properties.
Comparison with Similar Compounds
- Diethyl 2,3-diisobutylsuccinate
- Diethyl 2,3-diethylsuccinate
Comparison: Diethyl 2,3-diacetylsuccinate is unique due to the presence of acetyl groups, which impart distinct chemical properties compared to other diesters of succinic acid. For instance, diethyl 2,3-diisobutylsuccinate and diethyl 2,3-diethylsuccinate have different alkyl substituents, leading to variations in reactivity and applications .
Properties
IUPAC Name |
diethyl 2,3-diacetylbutanedioate | |
---|---|---|
Source | PubChem | |
URL | https://pubchem.ncbi.nlm.nih.gov | |
Description | Data deposited in or computed by PubChem | |
InChI |
InChI=1S/C12H18O6/c1-5-17-11(15)9(7(3)13)10(8(4)14)12(16)18-6-2/h9-10H,5-6H2,1-4H3 | |
Source | PubChem | |
URL | https://pubchem.ncbi.nlm.nih.gov | |
Description | Data deposited in or computed by PubChem | |
InChI Key |
GJEDSIHWCPPJFN-UHFFFAOYSA-N | |
Source | PubChem | |
URL | https://pubchem.ncbi.nlm.nih.gov | |
Description | Data deposited in or computed by PubChem | |
Canonical SMILES |
CCOC(=O)C(C(C(=O)C)C(=O)OCC)C(=O)C | |
Source | PubChem | |
URL | https://pubchem.ncbi.nlm.nih.gov | |
Description | Data deposited in or computed by PubChem | |
Molecular Formula |
C12H18O6 | |
Source | PubChem | |
URL | https://pubchem.ncbi.nlm.nih.gov | |
Description | Data deposited in or computed by PubChem | |
DSSTOX Substance ID |
DTXSID90883788 | |
Record name | Butanedioic acid, 2,3-diacetyl-, 1,4-diethyl ester | |
Source | EPA DSSTox | |
URL | https://comptox.epa.gov/dashboard/DTXSID90883788 | |
Description | DSSTox provides a high quality public chemistry resource for supporting improved predictive toxicology. | |
Molecular Weight |
258.27 g/mol | |
Source | PubChem | |
URL | https://pubchem.ncbi.nlm.nih.gov | |
Description | Data deposited in or computed by PubChem | |
CAS No. |
2049-86-7 | |
Record name | 1,4-Diethyl 2,3-diacetylbutanedioate | |
Source | CAS Common Chemistry | |
URL | https://commonchemistry.cas.org/detail?cas_rn=2049-86-7 | |
Description | CAS Common Chemistry is an open community resource for accessing chemical information. Nearly 500,000 chemical substances from CAS REGISTRY cover areas of community interest, including common and frequently regulated chemicals, and those relevant to high school and undergraduate chemistry classes. This chemical information, curated by our expert scientists, is provided in alignment with our mission as a division of the American Chemical Society. | |
Explanation | The data from CAS Common Chemistry is provided under a CC-BY-NC 4.0 license, unless otherwise stated. | |
Record name | 3,4-Dicarbethoxyhexane-2,5-dione | |
Source | ChemIDplus | |
URL | https://pubchem.ncbi.nlm.nih.gov/substance/?source=chemidplus&sourceid=0002049867 | |
Description | ChemIDplus is a free, web search system that provides access to the structure and nomenclature authority files used for the identification of chemical substances cited in National Library of Medicine (NLM) databases, including the TOXNET system. | |
Record name | Diethyl 2,3-diacetylsuccinate | |
Source | DTP/NCI | |
URL | https://dtp.cancer.gov/dtpstandard/servlet/dwindex?searchtype=NSC&outputformat=html&searchlist=6782 | |
Description | The NCI Development Therapeutics Program (DTP) provides services and resources to the academic and private-sector research communities worldwide to facilitate the discovery and development of new cancer therapeutic agents. | |
Explanation | Unless otherwise indicated, all text within NCI products is free of copyright and may be reused without our permission. Credit the National Cancer Institute as the source. | |
Record name | Butanedioic acid, 2,3-diacetyl-, 1,4-diethyl ester | |
Source | EPA Chemicals under the TSCA | |
URL | https://www.epa.gov/chemicals-under-tsca | |
Description | EPA Chemicals under the Toxic Substances Control Act (TSCA) collection contains information on chemicals and their regulations under TSCA, including non-confidential content from the TSCA Chemical Substance Inventory and Chemical Data Reporting. | |
Record name | Butanedioic acid, 2,3-diacetyl-, 1,4-diethyl ester | |
Source | EPA DSSTox | |
URL | https://comptox.epa.gov/dashboard/DTXSID90883788 | |
Description | DSSTox provides a high quality public chemistry resource for supporting improved predictive toxicology. | |
Record name | Diethyl 2,3-diacetylsuccinate | |
Source | European Chemicals Agency (ECHA) | |
URL | https://echa.europa.eu/substance-information/-/substanceinfo/100.016.432 | |
Description | The European Chemicals Agency (ECHA) is an agency of the European Union which is the driving force among regulatory authorities in implementing the EU's groundbreaking chemicals legislation for the benefit of human health and the environment as well as for innovation and competitiveness. | |
Explanation | Use of the information, documents and data from the ECHA website is subject to the terms and conditions of this Legal Notice, and subject to other binding limitations provided for under applicable law, the information, documents and data made available on the ECHA website may be reproduced, distributed and/or used, totally or in part, for non-commercial purposes provided that ECHA is acknowledged as the source: "Source: European Chemicals Agency, http://echa.europa.eu/". Such acknowledgement must be included in each copy of the material. ECHA permits and encourages organisations and individuals to create links to the ECHA website under the following cumulative conditions: Links can only be made to webpages that provide a link to the Legal Notice page. | |
Retrosynthesis Analysis
AI-Powered Synthesis Planning: Our tool employs the Template_relevance Pistachio, Template_relevance Bkms_metabolic, Template_relevance Pistachio_ringbreaker, Template_relevance Reaxys, Template_relevance Reaxys_biocatalysis model, leveraging a vast database of chemical reactions to predict feasible synthetic routes.
One-Step Synthesis Focus: Specifically designed for one-step synthesis, it provides concise and direct routes for your target compounds, streamlining the synthesis process.
Accurate Predictions: Utilizing the extensive PISTACHIO, BKMS_METABOLIC, PISTACHIO_RINGBREAKER, REAXYS, REAXYS_BIOCATALYSIS database, our tool offers high-accuracy predictions, reflecting the latest in chemical research and data.
Strategy Settings
Precursor scoring | Relevance Heuristic |
---|---|
Min. plausibility | 0.01 |
Model | Template_relevance |
Template Set | Pistachio/Bkms_metabolic/Pistachio_ringbreaker/Reaxys/Reaxys_biocatalysis |
Top-N result to add to graph | 6 |
Feasible Synthetic Routes
Q1: What is the significance of Diethyl 2,3-diacetylsuccinate in organic synthesis?
A: this compound is a versatile precursor in organic synthesis. It can be used to synthesize other valuable compounds. For example, it serves as a starting material for the preparation of Ethyl 2,5-dimethylfuran-3-carboxylate and 2,5-Dimethylfuran-3,4-dicarboxylic acid [, ].
Q2: Can this compound be formed as a byproduct in reactions involving other reagents?
A: Yes, research indicates that this compound can be an unexpected byproduct in certain reactions. For instance, in the transition metal salt-mediated oxidative radical addition of Ethyl acetoacetate to Limonene, this compound is observed alongside the desired dihydrofuran product, particularly when using Cerium(IV) ammonium nitrate (CAN) or Cobalt(II) acetate []. This highlights the importance of reaction optimization and understanding potential side reactions.
Q3: Are there alternative reagents to Manganese(III) acetate for the oxidative radical addition of Ethyl acetoacetate to Limonene?
A: While Manganese(III) acetate provides the highest yield (72%) of the desired dihydrofuran product in the oxidative radical addition of Ethyl acetoacetate to Limonene, other transition metal salts were also investigated []. Cerium(IV) ammonium nitrate (CAN) and Cobalt(II) acetate resulted in lower yields of the dihydrofuran product and also produced this compound as a byproduct. Iron(III) salts led to complex product mixtures, and Copper(II) chloride showed no reactivity. This suggests that the choice of transition metal salt significantly influences both the yield and selectivity of this reaction.
Disclaimer and Information on In-Vitro Research Products
Please be aware that all articles and product information presented on BenchChem are intended solely for informational purposes. The products available for purchase on BenchChem are specifically designed for in-vitro studies, which are conducted outside of living organisms. In-vitro studies, derived from the Latin term "in glass," involve experiments performed in controlled laboratory settings using cells or tissues. It is important to note that these products are not categorized as medicines or drugs, and they have not received approval from the FDA for the prevention, treatment, or cure of any medical condition, ailment, or disease. We must emphasize that any form of bodily introduction of these products into humans or animals is strictly prohibited by law. It is essential to adhere to these guidelines to ensure compliance with legal and ethical standards in research and experimentation.