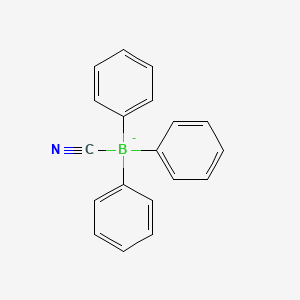
Cyanotriphenylborate
Overview
Description
Cyanotriphenylborate is an organoboron compound with the molecular formula C19H15BN. It is a tetraorganoborate ion, characterized by the presence of a cyanide group attached to a triphenylborate structure . This compound is known for its unique chemical properties and has been studied for various applications in scientific research.
Preparation Methods
Synthetic Routes and Reaction Conditions: Cyanotriphenylborate can be synthesized through several methods. One common approach involves the reaction of triphenylborane with cyanide sources under controlled conditions. For example, sodium cyanide can be used as a cyanide source in an organic solvent to facilitate the formation of this compound.
Industrial Production Methods: Industrial production of this compound typically involves large-scale synthesis using optimized reaction conditions to ensure high yield and purity. The process may include steps such as purification through recrystallization and solvent extraction to obtain the desired product.
Chemical Reactions Analysis
Types of Reactions: Cyanotriphenylborate undergoes various chemical reactions, including:
Substitution Reactions: The cyanide group can participate in nucleophilic substitution reactions, where it is replaced by other nucleophiles.
Coordination Reactions: this compound can form coordination complexes with metal ions, which are useful in catalysis and material science
Common Reagents and Conditions:
Nucleophiles: Common nucleophiles used in substitution reactions include halides, amines, and thiols.
Metal Ions: Transition metal ions such as palladium, platinum, and lanthanides are often used in coordination reactions.
Major Products:
Substitution Products: Depending on the nucleophile, various substituted borate compounds can be formed.
Coordination Complexes: Metal-cyanotriphenylborate complexes are formed, which have applications in catalysis and material science.
Scientific Research Applications
Cyanotriphenylborate has a wide range of applications in scientific research, including:
Mechanism of Action
Cyanotriphenylborate acts as a subtype-specific blocker of glycine receptor chloride channels. It binds to the chloride channel of the recombinant alpha 1-subunit homooligomeric glycine receptor, causing noncompetitive and use-dependent inhibition. This blockage is more pronounced at positive membrane potentials, suggesting an open-channel block mechanism. The sensitivity to this compound is influenced by a single glycine residue at position 254 of the alpha 1 subunit .
Comparison with Similar Compounds
Sodium cyanotriphenylborate: Similar in structure but includes a sodium ion.
Triphenylborane: Lacks the cyanide group, making it less reactive in certain substitution reactions.
Uniqueness: this compound’s unique combination of a cyanide group and triphenylborate structure allows it to participate in a variety of chemical reactions and form stable coordination complexes. Its ability to block glycine receptor chloride channels distinguishes it from other borate compounds and makes it valuable in neuropharmacology research .
Properties
IUPAC Name |
cyano(triphenyl)boranuide | |
---|---|---|
Details | Computed by Lexichem TK 2.7.0 (PubChem release 2021.10.14) | |
Source | PubChem | |
URL | https://pubchem.ncbi.nlm.nih.gov | |
Description | Data deposited in or computed by PubChem | |
InChI |
InChI=1S/C19H15BN/c21-16-20(17-10-4-1-5-11-17,18-12-6-2-7-13-18)19-14-8-3-9-15-19/h1-15H/q-1 | |
Details | Computed by InChI 1.0.6 (PubChem release 2021.10.14) | |
Source | PubChem | |
URL | https://pubchem.ncbi.nlm.nih.gov | |
Description | Data deposited in or computed by PubChem | |
InChI Key |
HYJITQQQMJSBOL-UHFFFAOYSA-N | |
Details | Computed by InChI 1.0.6 (PubChem release 2021.10.14) | |
Source | PubChem | |
URL | https://pubchem.ncbi.nlm.nih.gov | |
Description | Data deposited in or computed by PubChem | |
Canonical SMILES |
[B-](C#N)(C1=CC=CC=C1)(C2=CC=CC=C2)C3=CC=CC=C3 | |
Details | Computed by OEChem 2.3.0 (PubChem release 2021.10.14) | |
Source | PubChem | |
URL | https://pubchem.ncbi.nlm.nih.gov | |
Description | Data deposited in or computed by PubChem | |
Molecular Formula |
C19H15BN- | |
Details | Computed by PubChem 2.2 (PubChem release 2021.10.14) | |
Source | PubChem | |
URL | https://pubchem.ncbi.nlm.nih.gov | |
Description | Data deposited in or computed by PubChem | |
Molecular Weight |
268.1 g/mol | |
Details | Computed by PubChem 2.2 (PubChem release 2021.10.14) | |
Source | PubChem | |
URL | https://pubchem.ncbi.nlm.nih.gov | |
Description | Data deposited in or computed by PubChem | |
CAS No. |
47107-21-1 | |
Record name | (T-4)-(Cyano-κC)triphenylborate(1-) | |
Source | CAS Common Chemistry | |
URL | https://commonchemistry.cas.org/detail?cas_rn=47107-21-1 | |
Description | CAS Common Chemistry is an open community resource for accessing chemical information. Nearly 500,000 chemical substances from CAS REGISTRY cover areas of community interest, including common and frequently regulated chemicals, and those relevant to high school and undergraduate chemistry classes. This chemical information, curated by our expert scientists, is provided in alignment with our mission as a division of the American Chemical Society. | |
Explanation | The data from CAS Common Chemistry is provided under a CC-BY-NC 4.0 license, unless otherwise stated. | |
Retrosynthesis Analysis
AI-Powered Synthesis Planning: Our tool employs the Template_relevance Pistachio, Template_relevance Bkms_metabolic, Template_relevance Pistachio_ringbreaker, Template_relevance Reaxys, Template_relevance Reaxys_biocatalysis model, leveraging a vast database of chemical reactions to predict feasible synthetic routes.
One-Step Synthesis Focus: Specifically designed for one-step synthesis, it provides concise and direct routes for your target compounds, streamlining the synthesis process.
Accurate Predictions: Utilizing the extensive PISTACHIO, BKMS_METABOLIC, PISTACHIO_RINGBREAKER, REAXYS, REAXYS_BIOCATALYSIS database, our tool offers high-accuracy predictions, reflecting the latest in chemical research and data.
Strategy Settings
Precursor scoring | Relevance Heuristic |
---|---|
Min. plausibility | 0.01 |
Model | Template_relevance |
Template Set | Pistachio/Bkms_metabolic/Pistachio_ringbreaker/Reaxys/Reaxys_biocatalysis |
Top-N result to add to graph | 6 |
Feasible Synthetic Routes
Disclaimer and Information on In-Vitro Research Products
Please be aware that all articles and product information presented on BenchChem are intended solely for informational purposes. The products available for purchase on BenchChem are specifically designed for in-vitro studies, which are conducted outside of living organisms. In-vitro studies, derived from the Latin term "in glass," involve experiments performed in controlled laboratory settings using cells or tissues. It is important to note that these products are not categorized as medicines or drugs, and they have not received approval from the FDA for the prevention, treatment, or cure of any medical condition, ailment, or disease. We must emphasize that any form of bodily introduction of these products into humans or animals is strictly prohibited by law. It is essential to adhere to these guidelines to ensure compliance with legal and ethical standards in research and experimentation.