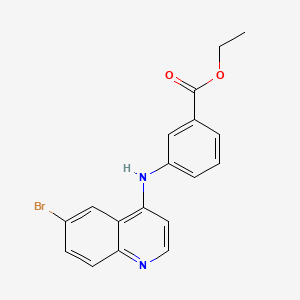
Ethyl 3-((6-bromoquinolin-4-yl)amino)benzoate
- Click on QUICK INQUIRY to receive a quote from our team of experts.
- With the quality product at a COMPETITIVE price, you can focus more on your research.
Overview
Description
Preparation Methods
Synthetic Routes and Reaction Conditions
The synthesis of Ethyl 3-((6-bromoquinolin-4-yl)amino)benzoate typically involves the Suzuki–Miyaura coupling reaction, which is a widely used method for forming carbon-carbon bonds . The reaction involves the coupling of an aryl halide with an organoboron compound in the presence of a palladium catalyst and a base . The general reaction conditions include:
Reagents: Aryl halide (6-bromoquinoline), organoboron compound (ethyl 3-aminobenzoate), palladium catalyst (e.g., Pd(PPh3)4), and a base (e.g., K2CO3).
Solvent: Typically, a polar aprotic solvent such as DMF or DMSO is used.
Temperature: The reaction is usually carried out at elevated temperatures, around 80-100°C.
Time: The reaction time can vary but is generally between 12-24 hours.
Industrial Production Methods
Industrial production of this compound follows similar synthetic routes but on a larger scale. The process involves optimizing reaction conditions to maximize yield and purity while minimizing costs and environmental impact. Continuous flow reactors and automated systems are often employed to enhance efficiency and scalability.
Chemical Reactions Analysis
Types of Reactions
Ethyl 3-((6-bromoquinolin-4-yl)amino)benzoate can undergo various chemical reactions, including:
Substitution Reactions: The bromine atom in the quinoline ring can be substituted with other nucleophiles under appropriate conditions.
Oxidation and Reduction: The compound can undergo oxidation and reduction reactions, altering the oxidation state of the nitrogen and carbon atoms in the quinoline ring.
Hydrolysis: The ester group can be hydrolyzed to form the corresponding carboxylic acid and alcohol.
Common Reagents and Conditions
Substitution: Nucleophiles such as amines or thiols in the presence of a base (e.g., NaOH) and a solvent (e.g., ethanol).
Oxidation: Oxidizing agents such as potassium permanganate (KMnO4) or chromium trioxide (CrO3) in acidic or basic conditions.
Reduction: Reducing agents such as sodium borohydride (NaBH4) or lithium aluminum hydride (LiAlH4) in anhydrous solvents.
Hydrolysis: Acidic or basic conditions using hydrochloric acid (HCl) or sodium hydroxide (NaOH) in water or alcohol.
Major Products
Substitution: Products depend on the nucleophile used, resulting in various substituted quinoline derivatives.
Oxidation: Oxidized quinoline derivatives with altered functional groups.
Reduction: Reduced quinoline derivatives with different oxidation states.
Hydrolysis: Carboxylic acid and alcohol.
Scientific Research Applications
Ethyl 3-((6-bromoquinolin-4-yl)amino)benzoate has several applications in scientific research, including:
Chemistry: Used as a building block in the synthesis of more complex organic molecules and heterocyclic compounds.
Biology: Investigated for its potential biological activities, including antimicrobial, antiviral, and anticancer properties.
Medicine: Explored as a lead compound in drug discovery and development for various therapeutic targets.
Industry: Utilized in the production of specialty chemicals and materials with specific properties.
Mechanism of Action
The mechanism of action of Ethyl 3-((6-bromoquinolin-4-yl)amino)benzoate involves its interaction with molecular targets and pathways in biological systems. The quinoline ring is known to intercalate with DNA, inhibiting the replication and transcription processes . Additionally, the compound may interact with enzymes and receptors, modulating their activity and leading to various biological effects .
Comparison with Similar Compounds
Ethyl 3-((6-bromoquinolin-4-yl)amino)benzoate can be compared with other quinoline derivatives, such as:
Chloroquine: An antimalarial drug with a similar quinoline structure but different substituents.
Quinoline: The parent compound with a simpler structure and diverse applications.
Quinacrine: Another antimalarial drug with additional biological activities.
The uniqueness of this compound lies in its specific substituents, which confer distinct chemical and biological properties compared to other quinoline derivatives .
Properties
Molecular Formula |
C18H15BrN2O2 |
---|---|
Molecular Weight |
371.2 g/mol |
IUPAC Name |
ethyl 3-[(6-bromoquinolin-4-yl)amino]benzoate |
InChI |
InChI=1S/C18H15BrN2O2/c1-2-23-18(22)12-4-3-5-14(10-12)21-17-8-9-20-16-7-6-13(19)11-15(16)17/h3-11H,2H2,1H3,(H,20,21) |
InChI Key |
KGSRGBKSNVWDAD-UHFFFAOYSA-N |
Canonical SMILES |
CCOC(=O)C1=CC(=CC=C1)NC2=C3C=C(C=CC3=NC=C2)Br |
Origin of Product |
United States |
Disclaimer and Information on In-Vitro Research Products
Please be aware that all articles and product information presented on BenchChem are intended solely for informational purposes. The products available for purchase on BenchChem are specifically designed for in-vitro studies, which are conducted outside of living organisms. In-vitro studies, derived from the Latin term "in glass," involve experiments performed in controlled laboratory settings using cells or tissues. It is important to note that these products are not categorized as medicines or drugs, and they have not received approval from the FDA for the prevention, treatment, or cure of any medical condition, ailment, or disease. We must emphasize that any form of bodily introduction of these products into humans or animals is strictly prohibited by law. It is essential to adhere to these guidelines to ensure compliance with legal and ethical standards in research and experimentation.