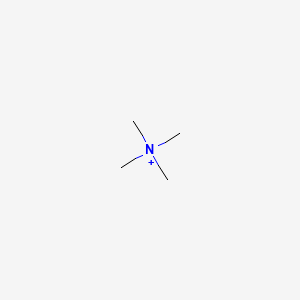
Tetramethylammonium
Overview
Description
Tetramethylammonium is the simplest quaternary ammonium cation with the chemical formula ( \text{C}4\text{H}{12}\text{N}^+ ). It consists of four methyl groups attached to a central nitrogen atom. This compound is isoelectronic with neopentane and is positively charged, typically isolated in association with a counter-ion. Common salts include this compound chloride and this compound hydroxide .
Mechanism of Action
Tetramethylammonium, also known as tetramethylazanium, is a simple quaternary ammonium cation with the chemical formula [Me4N]+ . It plays a significant role in various biochemical processes. This article will delve into the mechanism of action of this compound, covering its primary targets, mode of action, affected biochemical pathways, pharmacokinetics, results of action, and the influence of environmental factors on its action.
Target of Action
The primary target of this compound is the UDP-glucose 4-epimerase . This enzyme plays a crucial role in carbohydrate metabolism, specifically in the process of galactose conversion to glucose.
Mode of Action
The exact mode of action of this compound is still under investigation. It is known that this compound interacts with its target, udp-glucose 4-epimerase, affecting its function . The specifics of this interaction and the resulting changes are yet to be fully understood.
Result of Action
It is known that it can cause significant systemic toxicity . In severe cases, exposure to this compound can lead to respiratory failure and death .
Action Environment
The action, efficacy, and stability of this compound can be influenced by various environmental factors. For instance, the concentration of this compound can significantly impact its toxicity. Exposure to concentrations as low as 2.38% has resulted in potentially fatal symptoms within one hour .
Biochemical Analysis
Biochemical Properties
Tetramethylammonium plays a significant role in biochemical reactions, particularly in the modulation of neurotransmission. It interacts with nicotinic and muscarinic acetylcholine receptors, initially stimulating and then blocking neurotransmission in sympathetic and parasympathetic ganglia . This compound acts as an agonist at muscarinic receptors in post-ganglionic nerve endings in smooth muscles, cardiac muscle, and exocrine glands . Additionally, this compound inhibits TRPM7 channel currents in human T lymphocytes .
Cellular Effects
This compound has profound effects on various types of cells and cellular processes. It influences cell function by affecting cell signaling pathways, gene expression, and cellular metabolism. In human T lymphocytes, this compound inhibits TRPM7 channel currents, which play a crucial role in cellular magnesium homeostasis . This inhibition can impact cell signaling and metabolic processes. Furthermore, this compound’s interaction with acetylcholine receptors affects neurotransmission and muscle function .
Molecular Mechanism
The molecular mechanism of this compound involves its interaction with specific biomolecules and its ability to modulate enzyme activity. This compound binds to acetylcholine receptors, acting as an agonist and subsequently blocking neurotransmission . It also inhibits TRPM7 channel currents in a voltage-dependent manner, affecting ion transport and cellular signaling . These interactions highlight the compound’s role in modulating cellular functions at the molecular level.
Temporal Effects in Laboratory Settings
In laboratory settings, the effects of this compound can change over time. The compound’s stability and degradation are crucial factors in its long-term effects on cellular function. This compound hydroxide, a common form of the compound, can cause severe skin burns and systemic toxicity within minutes of exposure . The rapid onset of these effects underscores the importance of proper handling and safety precautions in laboratory environments.
Dosage Effects in Animal Models
The effects of this compound vary with different dosages in animal models. High doses of this compound can lead to toxic or adverse effects, including respiratory failure and death . In a rat model, dermal exposure to high concentrations of this compound hydroxide resulted in significant systemic toxicity and mortality . These findings highlight the importance of dosage control in experimental settings.
Metabolic Pathways
This compound is involved in various metabolic pathways, interacting with enzymes and cofactors. It catalyzes the reversible epimerization of UDP-glucose to UDP-galactose and UDP-N-acetylglucosamine to UDP-N-acetylgalactosamine . These reactions are essential for cellular metabolism and the synthesis of glycoproteins and glycolipids. This compound’s role in these pathways underscores its importance in cellular biochemistry.
Transport and Distribution
This compound is transported and distributed within cells and tissues through a combination of simple diffusion and carrier-mediated transport . Studies on the rat jejunum indicated nearly 100% absorption of this compound within 60 to 90 minutes . This efficient transport mechanism ensures the compound’s rapid distribution and accumulation in target tissues.
Subcellular Localization
The subcellular localization of this compound affects its activity and function. This compound chloride, a common form of the compound, destabilizes the tertiary structures of tRNAs and enhances the formation of tRNA-oligoDNA hybrids . This localization within the cellular environment influences the compound’s interactions with biomolecules and its overall biochemical activity.
Preparation Methods
Synthetic Routes and Reaction Conditions: Tetramethylammonium ion is typically prepared by the reaction between trimethylamine and a methyl chloride: [ \text{Me}_3\text{N} + \text{MeCl} \rightarrow \text{Me}_4\text{N}+\text{Cl}- ] This reaction is suitable for common halides. This compound salts with more complex anions may be prepared by salt metathesis reactions. For example, this compound borohydride can be made from this compound hydroxide .
Industrial Production Methods: One industrial method involves the reaction of trimethylamine with dimethyl carbonate to form this compound bicarbonate, which is then electrolyzed to produce this compound hydroxide. The resulting solution can be concentrated or diluted to obtain the desired concentration .
Chemical Reactions Analysis
Types of Reactions: Tetramethylammonium undergoes various chemical reactions, including:
Oxidation: this compound salts can be oxidized under specific conditions.
Reduction: Reduction reactions are less common but can occur under certain conditions.
Substitution: this compound salts can undergo nucleophilic substitution reactions.
Common Reagents and Conditions:
Oxidation: Strong oxidizing agents such as potassium permanganate.
Reduction: Reducing agents like lithium aluminum hydride.
Substitution: Nucleophiles such as hydroxide ions or halides.
Major Products:
Oxidation: Products depend on the specific oxidizing agent used.
Reduction: Reduced forms of the original compound.
Substitution: Substituted ammonium salts.
Scientific Research Applications
Tetramethylammonium has diverse applications in scientific research:
Chemistry: Used as a phase-transfer catalyst and in the synthesis of various organic compounds.
Biology: Employed in studies involving ion channels and neurotransmission.
Medicine: Investigated for its potential pharmacological effects.
Industry: Utilized in the production of semiconductors and as an etchant in microfabrication
Comparison with Similar Compounds
- Tetramethylammonium hydroxide
- Tetraethylammonium
- Tetrapropylammonium
Comparison: this compound is unique due to its high hydrophilicity and relatively simple structure. Compared to tetraethylammonium and tetrapropylammonium, it has a smaller molecular size, which influences its solubility and reactivity. This compound hydroxide is a stronger base than its tri- or dimethylamine counterparts due to the presence of the hydroxide ion .
Properties
IUPAC Name |
tetramethylazanium | |
---|---|---|
Source | PubChem | |
URL | https://pubchem.ncbi.nlm.nih.gov | |
Description | Data deposited in or computed by PubChem | |
InChI |
InChI=1S/C4H12N/c1-5(2,3)4/h1-4H3/q+1 | |
Source | PubChem | |
URL | https://pubchem.ncbi.nlm.nih.gov | |
Description | Data deposited in or computed by PubChem | |
InChI Key |
QEMXHQIAXOOASZ-UHFFFAOYSA-N | |
Source | PubChem | |
URL | https://pubchem.ncbi.nlm.nih.gov | |
Description | Data deposited in or computed by PubChem | |
Canonical SMILES |
C[N+](C)(C)C | |
Source | PubChem | |
URL | https://pubchem.ncbi.nlm.nih.gov | |
Description | Data deposited in or computed by PubChem | |
Molecular Formula |
C4H12N+ | |
Source | PubChem | |
URL | https://pubchem.ncbi.nlm.nih.gov | |
Description | Data deposited in or computed by PubChem | |
Related CAS |
14190-16-0 (sulfate[2:1]), 15625-56-6 (tribromide), 1941-24-8 (nitrate), 2537-36-2 (perchlorate), 373-68-2 (fluoride), 4337-68-2 (triiodide), 64-20-0 (bromide), 75-57-0 (chloride), 75-58-1 (iodide), 75-59-2 (hydroxide) | |
Record name | Tetramethylammonium | |
Source | ChemIDplus | |
URL | https://pubchem.ncbi.nlm.nih.gov/substance/?source=chemidplus&sourceid=0000051923 | |
Description | ChemIDplus is a free, web search system that provides access to the structure and nomenclature authority files used for the identification of chemical substances cited in National Library of Medicine (NLM) databases, including the TOXNET system. | |
DSSTOX Substance ID |
DTXSID3048071 | |
Record name | Tetramethylammonium | |
Source | EPA DSSTox | |
URL | https://comptox.epa.gov/dashboard/DTXSID3048071 | |
Description | DSSTox provides a high quality public chemistry resource for supporting improved predictive toxicology. | |
Molecular Weight |
74.14 g/mol | |
Source | PubChem | |
URL | https://pubchem.ncbi.nlm.nih.gov | |
Description | Data deposited in or computed by PubChem | |
CAS No. |
51-92-3, 19269-48-8 | |
Record name | Tetramethylammonium | |
Source | CAS Common Chemistry | |
URL | https://commonchemistry.cas.org/detail?cas_rn=51-92-3 | |
Description | CAS Common Chemistry is an open community resource for accessing chemical information. Nearly 500,000 chemical substances from CAS REGISTRY cover areas of community interest, including common and frequently regulated chemicals, and those relevant to high school and undergraduate chemistry classes. This chemical information, curated by our expert scientists, is provided in alignment with our mission as a division of the American Chemical Society. | |
Explanation | The data from CAS Common Chemistry is provided under a CC-BY-NC 4.0 license, unless otherwise stated. | |
Record name | Tetramethylammonium | |
Source | ChemIDplus | |
URL | https://pubchem.ncbi.nlm.nih.gov/substance/?source=chemidplus&sourceid=0000051923 | |
Description | ChemIDplus is a free, web search system that provides access to the structure and nomenclature authority files used for the identification of chemical substances cited in National Library of Medicine (NLM) databases, including the TOXNET system. | |
Record name | Tetramethylammonium | |
Source | DrugBank | |
URL | https://www.drugbank.ca/drugs/DB03095 | |
Description | The DrugBank database is a unique bioinformatics and cheminformatics resource that combines detailed drug (i.e. chemical, pharmacological and pharmaceutical) data with comprehensive drug target (i.e. sequence, structure, and pathway) information. | |
Explanation | Creative Common's Attribution-NonCommercial 4.0 International License (http://creativecommons.org/licenses/by-nc/4.0/legalcode) | |
Record name | Ammonium, (pentaiodide) | |
Source | DTP/NCI | |
URL | https://dtp.cancer.gov/dtpstandard/servlet/dwindex?searchtype=NSC&outputformat=html&searchlist=102944 | |
Description | The NCI Development Therapeutics Program (DTP) provides services and resources to the academic and private-sector research communities worldwide to facilitate the discovery and development of new cancer therapeutic agents. | |
Explanation | Unless otherwise indicated, all text within NCI products is free of copyright and may be reused without our permission. Credit the National Cancer Institute as the source. | |
Record name | Tetramethylammonium | |
Source | EPA DSSTox | |
URL | https://comptox.epa.gov/dashboard/DTXSID3048071 | |
Description | DSSTox provides a high quality public chemistry resource for supporting improved predictive toxicology. | |
Record name | TETRAMETHYLAMMONIUM | |
Source | FDA Global Substance Registration System (GSRS) | |
URL | https://gsrs.ncats.nih.gov/ginas/app/beta/substances/H0W55235FC | |
Description | The FDA Global Substance Registration System (GSRS) enables the efficient and accurate exchange of information on what substances are in regulated products. Instead of relying on names, which vary across regulatory domains, countries, and regions, the GSRS knowledge base makes it possible for substances to be defined by standardized, scientific descriptions. | |
Explanation | Unless otherwise noted, the contents of the FDA website (www.fda.gov), both text and graphics, are not copyrighted. They are in the public domain and may be republished, reprinted and otherwise used freely by anyone without the need to obtain permission from FDA. Credit to the U.S. Food and Drug Administration as the source is appreciated but not required. | |
Synthesis routes and methods
Procedure details
Retrosynthesis Analysis
AI-Powered Synthesis Planning: Our tool employs the Template_relevance Pistachio, Template_relevance Bkms_metabolic, Template_relevance Pistachio_ringbreaker, Template_relevance Reaxys, Template_relevance Reaxys_biocatalysis model, leveraging a vast database of chemical reactions to predict feasible synthetic routes.
One-Step Synthesis Focus: Specifically designed for one-step synthesis, it provides concise and direct routes for your target compounds, streamlining the synthesis process.
Accurate Predictions: Utilizing the extensive PISTACHIO, BKMS_METABOLIC, PISTACHIO_RINGBREAKER, REAXYS, REAXYS_BIOCATALYSIS database, our tool offers high-accuracy predictions, reflecting the latest in chemical research and data.
Strategy Settings
Precursor scoring | Relevance Heuristic |
---|---|
Min. plausibility | 0.01 |
Model | Template_relevance |
Template Set | Pistachio/Bkms_metabolic/Pistachio_ringbreaker/Reaxys/Reaxys_biocatalysis |
Top-N result to add to graph | 6 |
Feasible Synthetic Routes
Disclaimer and Information on In-Vitro Research Products
Please be aware that all articles and product information presented on BenchChem are intended solely for informational purposes. The products available for purchase on BenchChem are specifically designed for in-vitro studies, which are conducted outside of living organisms. In-vitro studies, derived from the Latin term "in glass," involve experiments performed in controlled laboratory settings using cells or tissues. It is important to note that these products are not categorized as medicines or drugs, and they have not received approval from the FDA for the prevention, treatment, or cure of any medical condition, ailment, or disease. We must emphasize that any form of bodily introduction of these products into humans or animals is strictly prohibited by law. It is essential to adhere to these guidelines to ensure compliance with legal and ethical standards in research and experimentation.