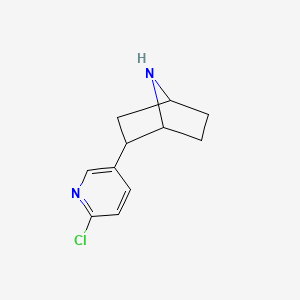
Epibatidine
Overview
Description
Epibatidine is a potent alkaloid that was first isolated from the skin of the Ecuadorian frog, Epipedobates anthonyi, in 1974 by John W. Daly . This compound is known for its powerful analgesic properties, which are significantly more potent than morphine. This compound’s structure was fully elucidated in 1992, revealing a chlorinated pyridine moiety connected to a 7-azabicyclo[2.2.1]heptane structure .
Mechanism of Action
Target of Action
Epibatidine primarily targets nicotinic acetylcholine receptors (nAChRs) , specifically the neuronal subtypes such as α4β2 and α7 . These receptors are crucial for neurotransmission in the central and peripheral nervous systems, playing roles in cognitive function, pain perception, and muscle contraction.
Mode of Action
This compound acts as an agonist at nAChRs . By binding to these receptors, it mimics the action of acetylcholine, leading to the opening of ion channels and subsequent influx of cations like sodium (Na+) and calcium (Ca2+). This depolarizes the neuron, triggering an action potential and neurotransmitter release .
Biochemical Pathways
The activation of nAChRs by this compound affects several biochemical pathways:
- Pain Pathways : this compound’s potent analgesic effects are due to its action on nAChRs in the pain pathways, reducing the perception of pain .
- Neurotransmitter Release : The influx of Ca2+ can lead to the release of various neurotransmitters, including dopamine, serotonin, and norepinephrine, influencing mood, arousal, and other functions .
Pharmacokinetics
This compound’s pharmacokinetics involve:
These properties contribute to its high bioavailability and potent effects, but also to its toxicity.
Result of Action
At the molecular level, this compound’s binding to nAChRs results in:
- Analgesia : Significant pain relief due to modulation of pain pathways .
- Toxicity : High doses can lead to overstimulation of nAChRs, causing muscle paralysis and respiratory failure .
Action Environment
Environmental factors influencing this compound’s action include:
- Diet : The presence of certain dietary components can influence its absorption and metabolism .
- Co-administered Drugs : Interactions with other drugs can alter its pharmacokinetics and dynamics .
This compound’s potent effects and narrow therapeutic index limit its therapeutic use, but it remains a valuable tool in neuropharmacological research.
Biochemical Analysis
Biochemical Properties
Epibatidine plays a crucial role in biochemical reactions by interacting with nicotinic acetylcholine receptors and muscarinic acetylcholine receptors. These receptors are integral to the transmission of nerve signals. This compound binds to the alpha-4 beta-2 subtype of nicotinic receptors with high affinity, which is believed to be responsible for its analgesic effects . Additionally, this compound interacts with muscarinic acetylcholine receptors, contributing to its overall pharmacological profile . The nature of these interactions involves the binding of this compound to the receptor sites, which modulates the receptor’s activity and influences downstream signaling pathways.
Cellular Effects
This compound exerts significant effects on various types of cells and cellular processes. In neuronal cells, this compound binding to nicotinic acetylcholine receptors leads to the opening of ion channels, allowing the influx of sodium and calcium ions. This depolarizes the cell membrane and propagates nerve signals . This compound also affects cell signaling pathways by modulating the activity of these receptors, which can influence gene expression and cellular metabolism. The compound’s interaction with muscarinic acetylcholine receptors can lead to changes in intracellular calcium levels, further impacting cellular functions .
Molecular Mechanism
The molecular mechanism of this compound involves its binding to nicotinic and muscarinic acetylcholine receptors. At the molecular level, this compound acts as an agonist for these receptors, mimicking the action of acetylcholine. By binding to the receptor sites, this compound induces a conformational change that opens the ion channels, allowing ion flow across the cell membrane . This action results in the depolarization of the neuron and the propagation of nerve impulses. Additionally, this compound’s binding to muscarinic receptors can activate G-protein coupled signaling pathways, leading to various cellular responses .
Temporal Effects in Laboratory Settings
In laboratory settings, the effects of this compound have been observed to change over time. This compound is relatively stable under controlled conditions, but its potency can decrease with prolonged exposure to light and air. Studies have shown that this compound can maintain its activity for extended periods when stored properly . Long-term exposure to this compound in in vitro studies has demonstrated sustained receptor activation, which can lead to receptor desensitization and downregulation . In vivo studies have indicated that repeated administration of this compound can result in tolerance, reducing its effectiveness over time .
Dosage Effects in Animal Models
The effects of this compound vary with different dosages in animal models. At low doses, this compound primarily affects nicotinic acetylcholine receptors, producing analgesic effects without significant toxicity . At higher doses, this compound can bind to muscarinic receptors, leading to adverse effects such as muscle paralysis and respiratory failure . The therapeutic window for this compound is narrow, with the effective dose being close to the lethal dose . Studies in animal models have shown that careful dosage management is crucial to harnessing the analgesic properties of this compound while minimizing its toxic effects .
Metabolic Pathways
This compound is metabolized primarily in the liver, where it undergoes biotransformation by cytochrome P450 enzymes . The metabolic pathways of this compound involve hydroxylation and conjugation reactions, leading to the formation of more water-soluble metabolites that can be excreted from the body . These metabolic processes are essential for the detoxification and elimination of this compound, ensuring that it does not accumulate to toxic levels . The interaction of this compound with metabolic enzymes can also influence its pharmacokinetics and overall bioavailability .
Transport and Distribution
Within cells and tissues, this compound is transported and distributed through various mechanisms. This compound can cross the blood-brain barrier, allowing it to exert its effects on central nervous system receptors . The compound is also distributed to peripheral tissues, where it interacts with nicotinic and muscarinic receptors. Transporters and binding proteins play a role in the cellular uptake and distribution of this compound, influencing its localization and accumulation within specific tissues . The distribution of this compound is critical for its pharmacological activity and therapeutic potential .
Subcellular Localization
This compound’s subcellular localization is primarily associated with its target receptors. The compound localizes to the cell membrane, where nicotinic and muscarinic acetylcholine receptors are situated . This localization is crucial for its activity, as it allows this compound to interact directly with its receptor targets. Additionally, this compound may undergo post-translational modifications that influence its targeting and function within specific cellular compartments . Understanding the subcellular localization of this compound is essential for elucidating its mechanism of action and optimizing its therapeutic applications .
Preparation Methods
Synthetic Routes and Reaction Conditions: Epibatidine can be synthesized through various methods. One notable approach involves the chemoenzymatic formal synthesis, which includes the enzymatic cis-dihydroxylation of bromobenzene using Pseudomonas putida UV4, followed by several steps to achieve the final product . Another method involves asymmetric desymmetrisation, asymmetric protonation, and Diels–Alder reactions with chiral allenes .
Industrial Production Methods: Industrial production of this compound is challenging due to its complex structure and the need for high stereoselectivity. preparative-scale methods have been developed, yielding both endo and exo isomers with reasonable efficiency .
Chemical Reactions Analysis
Types of Reactions: Epibatidine undergoes various chemical reactions, including oxidation, reduction, and substitution. These reactions are crucial for modifying its structure and enhancing its pharmacological properties.
Common Reagents and Conditions: Common reagents used in the synthesis and modification of this compound include palladium catalysts, chiral auxiliaries, and various organic solvents. Reaction conditions often involve controlled temperatures and pressures to ensure high yields and selectivity .
Major Products: The major products formed from these reactions are typically analogues of this compound with modified pharmacological profiles. These analogues are studied for their potential therapeutic applications and reduced toxicity .
Scientific Research Applications
Epibatidine has been extensively studied for its applications in various fields:
Chemistry: In chemistry, this compound serves as a model compound for studying the synthesis of complex alkaloids and the development of new synthetic methodologies .
Biology: In biological research, this compound is used to study the function of nicotinic acetylcholine receptors (nAChRs), which play a crucial role in neurotransmission .
Medicine: this compound’s potent analgesic properties have made it a subject of interest in pain management research. its high toxicity has limited its therapeutic use. Researchers are developing synthetic analogues with improved safety profiles .
Industry: In the pharmaceutical industry, this compound and its analogues are explored for their potential as new analgesic drugs. The compound’s unique structure and mechanism of action provide valuable insights for drug development .
Comparison with Similar Compounds
Epibatidine is unique due to its high potency and specific receptor interactions. Similar compounds include:
ABT-594: A synthetic analogue of this compound with improved safety and selectivity.
ABT-418: Another analogue with potential therapeutic applications.
Nicotine: Shares a similar mechanism of action but is less potent.
These compounds highlight the potential of this compound analogues in developing new analgesic drugs with better safety profiles.
Properties
IUPAC Name |
2-(6-chloropyridin-3-yl)-7-azabicyclo[2.2.1]heptane | |
---|---|---|
Source | PubChem | |
URL | https://pubchem.ncbi.nlm.nih.gov | |
Description | Data deposited in or computed by PubChem | |
InChI |
InChI=1S/C11H13ClN2/c12-11-4-1-7(6-13-11)9-5-8-2-3-10(9)14-8/h1,4,6,8-10,14H,2-3,5H2 | |
Source | PubChem | |
URL | https://pubchem.ncbi.nlm.nih.gov | |
Description | Data deposited in or computed by PubChem | |
InChI Key |
NLPRAJRHRHZCQQ-UHFFFAOYSA-N | |
Source | PubChem | |
URL | https://pubchem.ncbi.nlm.nih.gov | |
Description | Data deposited in or computed by PubChem | |
Canonical SMILES |
C1CC2C(CC1N2)C3=CN=C(C=C3)Cl | |
Source | PubChem | |
URL | https://pubchem.ncbi.nlm.nih.gov | |
Description | Data deposited in or computed by PubChem | |
Molecular Formula |
C11H13ClN2 | |
Source | PubChem | |
URL | https://pubchem.ncbi.nlm.nih.gov | |
Description | Data deposited in or computed by PubChem | |
DSSTOX Substance ID |
DTXSID30861409 | |
Record name | 2-(6-Chloropyridin-3-yl)-7-azabicyclo[2.2.1]heptane | |
Source | EPA DSSTox | |
URL | https://comptox.epa.gov/dashboard/DTXSID30861409 | |
Description | DSSTox provides a high quality public chemistry resource for supporting improved predictive toxicology. | |
Molecular Weight |
208.69 g/mol | |
Source | PubChem | |
URL | https://pubchem.ncbi.nlm.nih.gov | |
Description | Data deposited in or computed by PubChem | |
Synthesis routes and methods I
Procedure details
Synthesis routes and methods II
Procedure details
Retrosynthesis Analysis
AI-Powered Synthesis Planning: Our tool employs the Template_relevance Pistachio, Template_relevance Bkms_metabolic, Template_relevance Pistachio_ringbreaker, Template_relevance Reaxys, Template_relevance Reaxys_biocatalysis model, leveraging a vast database of chemical reactions to predict feasible synthetic routes.
One-Step Synthesis Focus: Specifically designed for one-step synthesis, it provides concise and direct routes for your target compounds, streamlining the synthesis process.
Accurate Predictions: Utilizing the extensive PISTACHIO, BKMS_METABOLIC, PISTACHIO_RINGBREAKER, REAXYS, REAXYS_BIOCATALYSIS database, our tool offers high-accuracy predictions, reflecting the latest in chemical research and data.
Strategy Settings
Precursor scoring | Relevance Heuristic |
---|---|
Min. plausibility | 0.01 |
Model | Template_relevance |
Template Set | Pistachio/Bkms_metabolic/Pistachio_ringbreaker/Reaxys/Reaxys_biocatalysis |
Top-N result to add to graph | 6 |
Feasible Synthetic Routes
Disclaimer and Information on In-Vitro Research Products
Please be aware that all articles and product information presented on BenchChem are intended solely for informational purposes. The products available for purchase on BenchChem are specifically designed for in-vitro studies, which are conducted outside of living organisms. In-vitro studies, derived from the Latin term "in glass," involve experiments performed in controlled laboratory settings using cells or tissues. It is important to note that these products are not categorized as medicines or drugs, and they have not received approval from the FDA for the prevention, treatment, or cure of any medical condition, ailment, or disease. We must emphasize that any form of bodily introduction of these products into humans or animals is strictly prohibited by law. It is essential to adhere to these guidelines to ensure compliance with legal and ethical standards in research and experimentation.