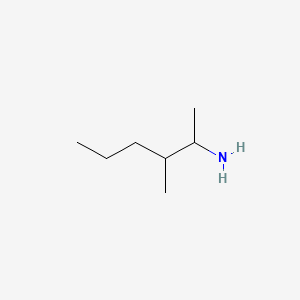
3-Methyl-2-hexylamine
- Click on QUICK INQUIRY to receive a quote from our team of experts.
- With the quality product at a COMPETITIVE price, you can focus more on your research.
Overview
Description
3-Methyl-2-hexanamine is an organic compound with the molecular formula C7H17N. It is a type of amine, specifically a secondary amine, characterized by the presence of a nitrogen atom bonded to two alkyl groups. This compound is also known by other names such as 3-methylhexan-2-amine and 2-hexanamine, 3-methyl-.
Preparation Methods
Synthetic Routes and Reaction Conditions
3-Methyl-2-hexanamine can be synthesized through several methods. One common approach involves the reaction of 3-methyl-2-hexanone with hydroxylamine to form an oxime, which is then reduced via catalytic hydrogenation to yield 3-methyl-2-hexanamine . Another method involves the reductive amination of 3-methyl-2-hexanone using ammonia and a reducing agent such as sodium cyanoborohydride.
Industrial Production Methods
In industrial settings, 3-methyl-2-hexanamine is often produced through the catalytic hydrogenation of 3-methyl-2-hexanone oxime. This process involves the use of a metal catalyst, such as palladium on carbon, under high pressure and temperature conditions to achieve efficient conversion.
Chemical Reactions Analysis
Types of Reactions
3-Methyl-2-hexanamine undergoes various chemical reactions, including:
Oxidation: This compound can be oxidized to form corresponding nitriles or amides under specific conditions.
Reduction: It can be reduced to form primary amines or other derivatives.
Substitution: 3-Methyl-2-hexanamine can participate in nucleophilic substitution reactions, where the amine group is replaced by other functional groups.
Common Reagents and Conditions
Oxidation: Reagents such as potassium permanganate or chromium trioxide can be used for oxidation reactions.
Reduction: Reducing agents like lithium aluminum hydride or sodium borohydride are commonly employed.
Substitution: Halogenating agents or alkylating agents are used in substitution reactions.
Major Products Formed
Oxidation: Nitriles or amides.
Reduction: Primary amines.
Substitution: Various substituted amines depending on the reagents used.
Scientific Research Applications
3-Methyl-2-hexanamine has several applications in scientific research:
Chemistry: It is used as a building block in organic synthesis and as an intermediate in the production of pharmaceuticals and agrochemicals.
Biology: This compound is studied for its potential biological activities, including its effects on neurotransmitter systems.
Medicine: It has been investigated for its potential use as a nasal decongestant and in the treatment of certain medical conditions.
Industry: 3-Methyl-2-hexanamine is used in the manufacture of various industrial chemicals and materials.
Mechanism of Action
The mechanism of action of 3-methyl-2-hexanamine involves its interaction with specific molecular targets and pathways. As an amine, it can act as a ligand for certain receptors, influencing neurotransmitter release and uptake. It may also interact with enzymes involved in metabolic pathways, altering their activity and leading to various physiological effects .
Comparison with Similar Compounds
Similar Compounds
2-Amino-4-methylhexane: Another similar compound with a different position of the amino group.
4-Methyl-2-hexanamine: Similar in structure but with a different arrangement of the methyl and amino groups.
Uniqueness
3-Methyl-2-hexanamine is unique due to its specific structure, which imparts distinct chemical and biological properties. Its position of the methyl and amino groups allows for unique interactions with molecular targets, making it valuable in various applications.
Properties
CAS No. |
35399-82-7 |
---|---|
Molecular Formula |
C7H17N |
Molecular Weight |
115.22 g/mol |
IUPAC Name |
3-methylhexan-2-amine |
InChI |
InChI=1S/C7H17N/c1-4-5-6(2)7(3)8/h6-7H,4-5,8H2,1-3H3 |
InChI Key |
WVYNIWHEHBSTSB-UHFFFAOYSA-N |
Canonical SMILES |
CCCC(C)C(C)N |
Origin of Product |
United States |
Disclaimer and Information on In-Vitro Research Products
Please be aware that all articles and product information presented on BenchChem are intended solely for informational purposes. The products available for purchase on BenchChem are specifically designed for in-vitro studies, which are conducted outside of living organisms. In-vitro studies, derived from the Latin term "in glass," involve experiments performed in controlled laboratory settings using cells or tissues. It is important to note that these products are not categorized as medicines or drugs, and they have not received approval from the FDA for the prevention, treatment, or cure of any medical condition, ailment, or disease. We must emphasize that any form of bodily introduction of these products into humans or animals is strictly prohibited by law. It is essential to adhere to these guidelines to ensure compliance with legal and ethical standards in research and experimentation.