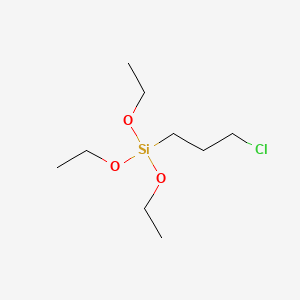
(3-Chloropropyl)triethoxysilane
Overview
Description
(3-Chloropropyl)triethoxysilane, also known as this compound, is a useful research compound. Its molecular formula is C9H21ClO3Si and its molecular weight is 240.8 g/mol. The purity is usually 95%.
The exact mass of the compound this compound is unknown and the complexity rating of the compound is unknown. The compound has been submitted to the National Cancer Institute (NCI) for testing and evaluation and the Cancer Chemotherapy National Service Center (NSC) number is 252156. The United Nations designated GHS hazard class pictogram is Flammable;Irritant, and the GHS signal word is WarningThe storage condition is unknown. Please store according to label instructions upon receipt of goods.
BenchChem offers high-quality this compound suitable for many research applications. Different packaging options are available to accommodate customers' requirements. Please inquire for more information about this compound including the price, delivery time, and more detailed information at info@benchchem.com.
Mechanism of Action
Target of Action
3-Chloropropyltriethoxysilane (CPTES) is an organosilane compound that primarily targets surfaces of various bio- and nano-materials . It is used to modify these surfaces, facilitating the attachment of other molecules for various applications .
Mode of Action
CPTES acts by forming a self-assembled monolayer (SAM) on the target surface . This SAM provides a platform for the covalent binding of other molecules to the surface .
Biochemical Pathways
The exact biochemical pathways affected by CPTES are dependent on the specific application and the molecules it is used to attach to the target surface. For example, in the case of surface modification of nanoparticles like carbon nanotubes (CNT) and silica nanoparticles (SiO2), CPTES can facilitate the development of molecularly imprinted polymers for protein detection and the fabrication of mesoporous silica .
Result of Action
The action of CPTES results in the successful modification of the target surface, enabling the attachment of other molecules for various applications . This can enhance the functionality of the surface, such as improving the sensitivity of biosensors or the efficacy of drug delivery systems .
Action Environment
The action of CPTES can be influenced by various environmental factors. For instance, the pH of the solution can affect the binding efficiency of CPTES to the target surface. Additionally, temperature and humidity can also impact the formation of the SAM and the subsequent surface modification process .
Properties
IUPAC Name |
3-chloropropyl(triethoxy)silane | |
---|---|---|
Source | PubChem | |
URL | https://pubchem.ncbi.nlm.nih.gov | |
Description | Data deposited in or computed by PubChem | |
InChI |
InChI=1S/C9H21ClO3Si/c1-4-11-14(12-5-2,13-6-3)9-7-8-10/h4-9H2,1-3H3 | |
Source | PubChem | |
URL | https://pubchem.ncbi.nlm.nih.gov | |
Description | Data deposited in or computed by PubChem | |
InChI Key |
KSCAZPYHLGGNPZ-UHFFFAOYSA-N | |
Source | PubChem | |
URL | https://pubchem.ncbi.nlm.nih.gov | |
Description | Data deposited in or computed by PubChem | |
Canonical SMILES |
CCO[Si](CCCCl)(OCC)OCC | |
Source | PubChem | |
URL | https://pubchem.ncbi.nlm.nih.gov | |
Description | Data deposited in or computed by PubChem | |
Molecular Formula |
C9H21ClO3Si | |
Source | PubChem | |
URL | https://pubchem.ncbi.nlm.nih.gov | |
Description | Data deposited in or computed by PubChem | |
DSSTOX Substance ID |
DTXSID5029267 | |
Record name | (3-Chloropropyl)(triethoxy)silane | |
Source | EPA DSSTox | |
URL | https://comptox.epa.gov/dashboard/DTXSID5029267 | |
Description | DSSTox provides a high quality public chemistry resource for supporting improved predictive toxicology. | |
Molecular Weight |
240.80 g/mol | |
Source | PubChem | |
URL | https://pubchem.ncbi.nlm.nih.gov | |
Description | Data deposited in or computed by PubChem | |
Physical Description |
Liquid; Pellets or Large Crystals, Liquid, Clear liquid; [Gelest MSDS] | |
Record name | Silane, (3-chloropropyl)triethoxy- | |
Source | EPA Chemicals under the TSCA | |
URL | https://www.epa.gov/chemicals-under-tsca | |
Description | EPA Chemicals under the Toxic Substances Control Act (TSCA) collection contains information on chemicals and their regulations under TSCA, including non-confidential content from the TSCA Chemical Substance Inventory and Chemical Data Reporting. | |
Record name | (3-Chloropropyl)triethoxysilane | |
Source | Haz-Map, Information on Hazardous Chemicals and Occupational Diseases | |
URL | https://haz-map.com/Agents/19109 | |
Description | Haz-Map® is an occupational health database designed for health and safety professionals and for consumers seeking information about the adverse effects of workplace exposures to chemical and biological agents. | |
Explanation | Copyright (c) 2022 Haz-Map(R). All rights reserved. Unless otherwise indicated, all materials from Haz-Map are copyrighted by Haz-Map(R). No part of these materials, either text or image may be used for any purpose other than for personal use. Therefore, reproduction, modification, storage in a retrieval system or retransmission, in any form or by any means, electronic, mechanical or otherwise, for reasons other than personal use, is strictly prohibited without prior written permission. | |
CAS No. |
5089-70-3, 29656-55-1 | |
Record name | (3-Chloropropyl)triethoxysilane | |
Source | CAS Common Chemistry | |
URL | https://commonchemistry.cas.org/detail?cas_rn=5089-70-3 | |
Description | CAS Common Chemistry is an open community resource for accessing chemical information. Nearly 500,000 chemical substances from CAS REGISTRY cover areas of community interest, including common and frequently regulated chemicals, and those relevant to high school and undergraduate chemistry classes. This chemical information, curated by our expert scientists, is provided in alignment with our mission as a division of the American Chemical Society. | |
Explanation | The data from CAS Common Chemistry is provided under a CC-BY-NC 4.0 license, unless otherwise stated. | |
Record name | (3-Chloropropyl)(triethoxy)silane | |
Source | ChemIDplus | |
URL | https://pubchem.ncbi.nlm.nih.gov/substance/?source=chemidplus&sourceid=0005089703 | |
Description | ChemIDplus is a free, web search system that provides access to the structure and nomenclature authority files used for the identification of chemical substances cited in National Library of Medicine (NLM) databases, including the TOXNET system. | |
Record name | (Chloropropyl)triethoxysilane | |
Source | ChemIDplus | |
URL | https://pubchem.ncbi.nlm.nih.gov/substance/?source=chemidplus&sourceid=0029656551 | |
Description | ChemIDplus is a free, web search system that provides access to the structure and nomenclature authority files used for the identification of chemical substances cited in National Library of Medicine (NLM) databases, including the TOXNET system. | |
Record name | (3-Chloropropyl)triethoxysilane | |
Source | DTP/NCI | |
URL | https://dtp.cancer.gov/dtpstandard/servlet/dwindex?searchtype=NSC&outputformat=html&searchlist=252156 | |
Description | The NCI Development Therapeutics Program (DTP) provides services and resources to the academic and private-sector research communities worldwide to facilitate the discovery and development of new cancer therapeutic agents. | |
Explanation | Unless otherwise indicated, all text within NCI products is free of copyright and may be reused without our permission. Credit the National Cancer Institute as the source. | |
Record name | Silane, (3-chloropropyl)triethoxy- | |
Source | EPA Chemicals under the TSCA | |
URL | https://www.epa.gov/chemicals-under-tsca | |
Description | EPA Chemicals under the Toxic Substances Control Act (TSCA) collection contains information on chemicals and their regulations under TSCA, including non-confidential content from the TSCA Chemical Substance Inventory and Chemical Data Reporting. | |
Record name | (3-Chloropropyl)(triethoxy)silane | |
Source | EPA DSSTox | |
URL | https://comptox.epa.gov/dashboard/DTXSID5029267 | |
Description | DSSTox provides a high quality public chemistry resource for supporting improved predictive toxicology. | |
Record name | (3-chloropropyl)triethoxysilane | |
Source | European Chemicals Agency (ECHA) | |
URL | https://echa.europa.eu/substance-information/-/substanceinfo/100.023.459 | |
Description | The European Chemicals Agency (ECHA) is an agency of the European Union which is the driving force among regulatory authorities in implementing the EU's groundbreaking chemicals legislation for the benefit of human health and the environment as well as for innovation and competitiveness. | |
Explanation | Use of the information, documents and data from the ECHA website is subject to the terms and conditions of this Legal Notice, and subject to other binding limitations provided for under applicable law, the information, documents and data made available on the ECHA website may be reproduced, distributed and/or used, totally or in part, for non-commercial purposes provided that ECHA is acknowledged as the source: "Source: European Chemicals Agency, http://echa.europa.eu/". Such acknowledgement must be included in each copy of the material. ECHA permits and encourages organisations and individuals to create links to the ECHA website under the following cumulative conditions: Links can only be made to webpages that provide a link to the Legal Notice page. | |
Record name | (chloropropyl)triethoxysilane | |
Source | European Chemicals Agency (ECHA) | |
URL | https://echa.europa.eu/substance-information/-/substanceinfo/100.045.216 | |
Description | The European Chemicals Agency (ECHA) is an agency of the European Union which is the driving force among regulatory authorities in implementing the EU's groundbreaking chemicals legislation for the benefit of human health and the environment as well as for innovation and competitiveness. | |
Explanation | Use of the information, documents and data from the ECHA website is subject to the terms and conditions of this Legal Notice, and subject to other binding limitations provided for under applicable law, the information, documents and data made available on the ECHA website may be reproduced, distributed and/or used, totally or in part, for non-commercial purposes provided that ECHA is acknowledged as the source: "Source: European Chemicals Agency, http://echa.europa.eu/". Such acknowledgement must be included in each copy of the material. ECHA permits and encourages organisations and individuals to create links to the ECHA website under the following cumulative conditions: Links can only be made to webpages that provide a link to the Legal Notice page. | |
Record name | (3-CHLOROPROPYL)(TRIETHOXY)SILANE | |
Source | FDA Global Substance Registration System (GSRS) | |
URL | https://gsrs.ncats.nih.gov/ginas/app/beta/substances/X7RB20518M | |
Description | The FDA Global Substance Registration System (GSRS) enables the efficient and accurate exchange of information on what substances are in regulated products. Instead of relying on names, which vary across regulatory domains, countries, and regions, the GSRS knowledge base makes it possible for substances to be defined by standardized, scientific descriptions. | |
Explanation | Unless otherwise noted, the contents of the FDA website (www.fda.gov), both text and graphics, are not copyrighted. They are in the public domain and may be republished, reprinted and otherwise used freely by anyone without the need to obtain permission from FDA. Credit to the U.S. Food and Drug Administration as the source is appreciated but not required. | |
Synthesis routes and methods I
Procedure details
Synthesis routes and methods II
Procedure details
Synthesis routes and methods III
Procedure details
Synthesis routes and methods IV
Procedure details
Retrosynthesis Analysis
AI-Powered Synthesis Planning: Our tool employs the Template_relevance Pistachio, Template_relevance Bkms_metabolic, Template_relevance Pistachio_ringbreaker, Template_relevance Reaxys, Template_relevance Reaxys_biocatalysis model, leveraging a vast database of chemical reactions to predict feasible synthetic routes.
One-Step Synthesis Focus: Specifically designed for one-step synthesis, it provides concise and direct routes for your target compounds, streamlining the synthesis process.
Accurate Predictions: Utilizing the extensive PISTACHIO, BKMS_METABOLIC, PISTACHIO_RINGBREAKER, REAXYS, REAXYS_BIOCATALYSIS database, our tool offers high-accuracy predictions, reflecting the latest in chemical research and data.
Strategy Settings
Precursor scoring | Relevance Heuristic |
---|---|
Min. plausibility | 0.01 |
Model | Template_relevance |
Template Set | Pistachio/Bkms_metabolic/Pistachio_ringbreaker/Reaxys/Reaxys_biocatalysis |
Top-N result to add to graph | 6 |
Feasible Synthetic Routes
A: (3-Chloropropyl)triethoxysilane primarily interacts with surfaces containing hydroxyl groups, like silica, through hydrolysis and condensation reactions. [, , ] This leads to the covalent attachment of the silane molecule to the surface, forming a stable siloxane (Si-O-Si) bond. [, ] This surface modification can impart various properties like hydrophobicity, altered surface charge, and provide anchor points for further functionalization with other molecules. [, , ]
ANone:
- Spectroscopic Data:
- FTIR: Characteristic peaks for Si-O-C (around 1100 cm-1), Si-C (around 800 cm-1), and C-Cl (around 700 cm-1) bonds are observed. [, , ]
- NMR: 1H and 13C NMR spectra can provide information on the different proton and carbon environments within the molecule. [, ] 29Si NMR is particularly useful for analyzing the condensation state of the siloxane network. []
A: this compound exhibits good compatibility with various materials like silica, alumina, and polymers. [, , ] It forms stable bonds with these materials, enhancing their hydrophobicity and modifying their surface properties. [, ] The compound is relatively stable under ambient conditions but undergoes hydrolysis in the presence of moisture. [] This hydrolysis is accelerated under acidic or basic conditions, leading to the formation of silanol groups and subsequent condensation reactions. []
A: While this compound itself is not a catalyst, it is widely used as a precursor for synthesizing heterogeneous catalysts. [, ] The chloro group serves as a reactive site for introducing various functional groups, like amines, thiols, or imidazoles, which can act as ligands for metal complexation. [, , ] These functionalized materials can then be used as catalysts in various reactions, offering advantages like easy separation, recyclability, and enhanced activity and selectivity. [, ]
A: Computational techniques like molecular dynamics simulations and density functional theory calculations are used to study the interaction of this compound with different surfaces at the molecular level. [] These simulations provide insights into the adsorption mechanism, orientation of molecules on the surface, and the effect of different functional groups on surface properties. [] Furthermore, QSAR models can be developed to predict the activity and properties of silane derivatives based on their molecular structure. []
A: Modifications to the structure of this compound, particularly to the chloropropyl group, can significantly impact its reactivity and downstream applications. [] For example, substituting the chlorine atom with other functional groups like amine, thiol, or azide can modify its reactivity towards nucleophiles and influence its ability to form specific chemical bonds. [, ] Furthermore, altering the length of the alkyl chain between the silicon and chlorine atoms can affect the hydrophobicity and steric hindrance of the molecule, influencing its interaction with surfaces and other molecules. []
A: this compound is susceptible to hydrolysis in the presence of moisture; therefore, it is typically stored under anhydrous conditions. [] Formulation strategies can involve utilizing suitable solvents, adding stabilizers, or encapsulating the compound to protect it from moisture and extend its shelf life. []
A: this compound should be handled with care as it can cause skin and eye irritation. [] Appropriate personal protective equipment, including gloves and eye protection, should be worn during handling. It is essential to consult the Safety Data Sheet (SDS) for detailed safety information and handling procedures. []
A: Analytical methods like gas chromatography (GC) and liquid chromatography (LC), coupled with suitable detectors, are commonly employed for characterizing and quantifying this compound. [] Method validation involves assessing parameters like accuracy, precision, linearity, limit of detection, and limit of quantification to ensure the reliability and robustness of the analytical data. []
A: Manufacturers of this compound typically implement strict quality control measures to ensure the purity, consistency, and quality of the product. [] These measures may include raw material testing, in-process controls during synthesis, and final product analysis to meet specified standards.
ANone: Research on this compound benefits from a wide range of infrastructure and resources available for material synthesis, characterization, and application testing. These include:
A: The use of silane coupling agents, including this compound, for surface modification gained prominence in the mid-20th century with the development of new materials and composites. [] Early research focused on their application in improving the adhesion between inorganic materials and polymers, leading to the development of stronger and more durable composites. Over the years, the applications of this compound have expanded to diverse areas, including catalysis, sensing, drug delivery, and nanotechnology.
Disclaimer and Information on In-Vitro Research Products
Please be aware that all articles and product information presented on BenchChem are intended solely for informational purposes. The products available for purchase on BenchChem are specifically designed for in-vitro studies, which are conducted outside of living organisms. In-vitro studies, derived from the Latin term "in glass," involve experiments performed in controlled laboratory settings using cells or tissues. It is important to note that these products are not categorized as medicines or drugs, and they have not received approval from the FDA for the prevention, treatment, or cure of any medical condition, ailment, or disease. We must emphasize that any form of bodily introduction of these products into humans or animals is strictly prohibited by law. It is essential to adhere to these guidelines to ensure compliance with legal and ethical standards in research and experimentation.