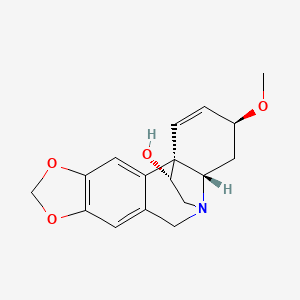
Haemanthamine
Overview
Description
Haemanthamine is a naturally occurring alkaloid found in plants of the Amaryllidaceae family. This compound is known for its unique 5,10b-ethanophenanthridine skeleton and has been extensively studied for its diverse biological activities, including antitumor, antimicrobial, and antiviral properties .
Mechanism of Action
Haemanthamine, also known as Haemanthamin, is a structurally diverse alkaloid derived from the Amaryllidaceae family of plants . This compound has gained considerable attention due to its wide range of biological properties, including antitumor properties .
Target of Action
this compound primarily targets the peptidyl transferase center of the eukaryotic ribosome . This center plays a crucial role in protein synthesis, making it a significant target for the compound’s action .
Mode of Action
this compound binds to the peptidyl transferase center of the eukaryotic ribosome, inhibiting protein synthesis . The compound’s specific conformational structure, including its C11 hydroxyl, C3 methoxy groups, and ring C1–C10b and C1–C2 bonds, are key to its interaction with its targets .
Biochemical Pathways
this compound affects the biochemical pathway of ribosome biogenesis. It increases nucleolar stress by inhibiting the early stages of ribosome biogenesis, leading to the activation of a p53-dependent antitumor pathway . The pathway from phenylalanine to 3,4-DHBA is also involved in the synthesis of this compound .
Pharmacokinetics
The pharmacokinetics of a compound greatly influence its bioavailability and efficacy .
Result of Action
this compound can trigger apoptosis in many cell lines, including A2780 ovarian cancer cells, as well as in p53-negative T cell leukemia Jurkat cells by activating caspases . It has shown strong growth inhibitory and cytotoxic activity against a wide range of cancer cell lines of different histotypes .
Biochemical Analysis
Biochemical Properties
Haemanthamine plays a crucial role in various biochemical reactions. It interacts with several enzymes, proteins, and other biomolecules, influencing their activity and function. One of the primary interactions of this compound is with ribosomes, where it binds to the peptidyl transferase center of the 60S ribosomal subunit . This binding inhibits protein synthesis by preventing the formation of peptide bonds, thereby exerting its antitumor effects . Additionally, this compound has been shown to interact with enzymes involved in the norbelladine pathway, which is responsible for the biosynthesis of Amaryllidaceae alkaloids .
Cellular Effects
This compound exerts significant effects on various types of cells and cellular processes. It has been demonstrated to inhibit the growth of cancer cells by blocking protein synthesis at the ribosomal level . This inhibition leads to nucleolar stress and the activation of a p53-dependent antitumor pathway, resulting in the stabilization of the protein p53 and the elimination of cancer cells . Furthermore, this compound influences cell signaling pathways, gene expression, and cellular metabolism, contributing to its broad-spectrum biological activities .
Molecular Mechanism
The molecular mechanism of this compound involves its binding interactions with biomolecules, enzyme inhibition, and changes in gene expression. This compound binds to the peptidyl transferase center of the eukaryotic ribosome, inhibiting protein synthesis by preventing peptide bond formation . This interaction disrupts the elongation phase of protein synthesis, leading to the inhibition of cancer cell growth . Additionally, this compound induces nucleolar stress, which activates the p53-dependent antitumor pathway and stabilizes the protein p53 .
Temporal Effects in Laboratory Settings
In laboratory settings, the effects of this compound have been observed to change over time. This compound increases nucleolar stress by inhibiting early stages of ribosome biogenesis, leading to the activation of a p53-dependent antitumor pathway . Over time, this inhibition of ribosome biogenesis results in the sustained activation of the antitumor pathway and the continued elimination of cancer cells . The stability and degradation of this compound in laboratory settings have also been studied, with findings indicating that it remains stable under various conditions .
Dosage Effects in Animal Models
The effects of this compound vary with different dosages in animal models. At lower doses, this compound has been shown to inhibit cancer cell proliferation and induce apoptosis without significant toxicity . At higher doses, this compound can cause adverse effects, including cytotoxicity and inhibition of normal cell growth . These dosage-dependent effects highlight the importance of optimizing the dosage of this compound for therapeutic applications .
Metabolic Pathways
This compound is involved in several metabolic pathways, including the norbelladine pathway, which is responsible for the biosynthesis of Amaryllidaceae alkaloids . This pathway involves the condensation of aromatic amino acid derivatives, such as 3,4-dihydroxybenzaldehyde and tyramine, to form 4’-O-methylnorbelladine, a key intermediate in the biosynthesis of this compound . This compound also interacts with enzymes and cofactors involved in these metabolic pathways, influencing metabolic flux and metabolite levels .
Transport and Distribution
This compound is transported and distributed within cells and tissues through interactions with transporters and binding proteins . These interactions facilitate the localization and accumulation of this compound in specific cellular compartments, where it exerts its biological effects . The distribution of this compound within tissues is influenced by its binding affinity to various biomolecules, which determines its localization and activity .
Subcellular Localization
The subcellular localization of this compound is primarily within the nucleolus, where it binds to ribosomes and inhibits protein synthesis . This localization is facilitated by targeting signals and post-translational modifications that direct this compound to specific compartments within the cell . The activity and function of this compound are influenced by its subcellular localization, as it exerts its effects primarily within the nucleolus .
Preparation Methods
Synthetic Routes and Reaction Conditions: The synthesis of haemanthamine involves several steps, starting from the condensation of aromatic amino acid derivatives such as 3,4-dihydroxybenzaldehyde and tyramine. The intermediate 4’-O-methylnorbelladine undergoes a series of reactions, including oxidative coupling, to form the characteristic 5,10b-ethanophenanthridine skeleton .
Industrial Production Methods: Industrial production of this compound is typically achieved through the extraction from Amaryllidaceae plants. Advanced techniques such as high-performance liquid chromatography (HPLC) are used to isolate and purify the compound from plant extracts .
Chemical Reactions Analysis
Types of Reactions: Haemanthamine undergoes various chemical reactions, including:
Oxidation: this compound can be oxidized to form different derivatives.
Reduction: Reduction reactions can modify the functional groups on the this compound molecule.
Substitution: Substitution reactions can introduce new functional groups into the this compound structure
Common Reagents and Conditions:
Oxidation: Common oxidizing agents include potassium permanganate and hydrogen peroxide.
Reduction: Reducing agents such as sodium borohydride are often used.
Substitution: Reagents like alkyl halides and acids are employed in substitution reactions
Major Products: The major products formed from these reactions include various this compound derivatives, which are studied for their enhanced biological activities .
Scientific Research Applications
Haemanthamine has a wide range of scientific research applications:
Chemistry: Used as a model compound for studying synthetic methodologies.
Biology: Investigated for its role in inhibiting ribosome biogenesis and inducing nucleolar stress.
Medicine: Explored for its potential as an antitumor agent due to its ability to activate p53-dependent pathways.
Industry: Utilized in the development of new pharmaceuticals and chemical probes .
Comparison with Similar Compounds
Haemanthamine is structurally similar to other Amaryllidaceae alkaloids such as haemanthidine and crinine. this compound is unique due to its specific 5,10b-ethanophenanthridine skeleton, which imparts distinct biological activities . Similar compounds include:
- Haemanthidine
- Crinine
- Lycorine
- Galanthamine .
This compound’s unique structure and diverse biological activities make it a compound of significant interest in various fields of scientific research.
Properties
IUPAC Name |
(1S,13S,15S,18R)-15-methoxy-5,7-dioxa-12-azapentacyclo[10.5.2.01,13.02,10.04,8]nonadeca-2,4(8),9,16-tetraen-18-ol | |
---|---|---|
Source | PubChem | |
URL | https://pubchem.ncbi.nlm.nih.gov | |
Description | Data deposited in or computed by PubChem | |
InChI |
InChI=1S/C17H19NO4/c1-20-11-2-3-17-12-6-14-13(21-9-22-14)4-10(12)7-18(8-16(17)19)15(17)5-11/h2-4,6,11,15-16,19H,5,7-9H2,1H3/t11-,15+,16+,17+/m1/s1 | |
Source | PubChem | |
URL | https://pubchem.ncbi.nlm.nih.gov | |
Description | Data deposited in or computed by PubChem | |
InChI Key |
YGPRSGKVLATIHT-HSHDSVGOSA-N | |
Source | PubChem | |
URL | https://pubchem.ncbi.nlm.nih.gov | |
Description | Data deposited in or computed by PubChem | |
Canonical SMILES |
COC1CC2C3(C=C1)C(CN2CC4=CC5=C(C=C34)OCO5)O | |
Source | PubChem | |
URL | https://pubchem.ncbi.nlm.nih.gov | |
Description | Data deposited in or computed by PubChem | |
Isomeric SMILES |
CO[C@H]1C[C@H]2[C@@]3(C=C1)[C@H](CN2CC4=CC5=C(C=C34)OCO5)O | |
Source | PubChem | |
URL | https://pubchem.ncbi.nlm.nih.gov | |
Description | Data deposited in or computed by PubChem | |
Molecular Formula |
C17H19NO4 | |
Source | PubChem | |
URL | https://pubchem.ncbi.nlm.nih.gov | |
Description | Data deposited in or computed by PubChem | |
DSSTOX Substance ID |
DTXSID30963614 | |
Record name | Haemanthamine | |
Source | EPA DSSTox | |
URL | https://comptox.epa.gov/dashboard/DTXSID30963614 | |
Description | DSSTox provides a high quality public chemistry resource for supporting improved predictive toxicology. | |
Molecular Weight |
301.34 g/mol | |
Source | PubChem | |
URL | https://pubchem.ncbi.nlm.nih.gov | |
Description | Data deposited in or computed by PubChem | |
CAS No. |
466-75-1 | |
Record name | (+)-Haemanthamine | |
Source | CAS Common Chemistry | |
URL | https://commonchemistry.cas.org/detail?cas_rn=466-75-1 | |
Description | CAS Common Chemistry is an open community resource for accessing chemical information. Nearly 500,000 chemical substances from CAS REGISTRY cover areas of community interest, including common and frequently regulated chemicals, and those relevant to high school and undergraduate chemistry classes. This chemical information, curated by our expert scientists, is provided in alignment with our mission as a division of the American Chemical Society. | |
Explanation | The data from CAS Common Chemistry is provided under a CC-BY-NC 4.0 license, unless otherwise stated. | |
Record name | Haemanthamine | |
Source | DTP/NCI | |
URL | https://dtp.cancer.gov/dtpstandard/servlet/dwindex?searchtype=NSC&outputformat=html&searchlist=403140 | |
Description | The NCI Development Therapeutics Program (DTP) provides services and resources to the academic and private-sector research communities worldwide to facilitate the discovery and development of new cancer therapeutic agents. | |
Explanation | Unless otherwise indicated, all text within NCI products is free of copyright and may be reused without our permission. Credit the National Cancer Institute as the source. | |
Record name | Haemanthamine | |
Source | EPA DSSTox | |
URL | https://comptox.epa.gov/dashboard/DTXSID30963614 | |
Description | DSSTox provides a high quality public chemistry resource for supporting improved predictive toxicology. | |
Record name | Hemeanthamin | |
Source | FDA Global Substance Registration System (GSRS) | |
URL | https://gsrs.ncats.nih.gov/ginas/app/beta/substances/VBL6HZX2ZB | |
Description | The FDA Global Substance Registration System (GSRS) enables the efficient and accurate exchange of information on what substances are in regulated products. Instead of relying on names, which vary across regulatory domains, countries, and regions, the GSRS knowledge base makes it possible for substances to be defined by standardized, scientific descriptions. | |
Explanation | Unless otherwise noted, the contents of the FDA website (www.fda.gov), both text and graphics, are not copyrighted. They are in the public domain and may be republished, reprinted and otherwise used freely by anyone without the need to obtain permission from FDA. Credit to the U.S. Food and Drug Administration as the source is appreciated but not required. | |
Retrosynthesis Analysis
AI-Powered Synthesis Planning: Our tool employs the Template_relevance Pistachio, Template_relevance Bkms_metabolic, Template_relevance Pistachio_ringbreaker, Template_relevance Reaxys, Template_relevance Reaxys_biocatalysis model, leveraging a vast database of chemical reactions to predict feasible synthetic routes.
One-Step Synthesis Focus: Specifically designed for one-step synthesis, it provides concise and direct routes for your target compounds, streamlining the synthesis process.
Accurate Predictions: Utilizing the extensive PISTACHIO, BKMS_METABOLIC, PISTACHIO_RINGBREAKER, REAXYS, REAXYS_BIOCATALYSIS database, our tool offers high-accuracy predictions, reflecting the latest in chemical research and data.
Strategy Settings
Precursor scoring | Relevance Heuristic |
---|---|
Min. plausibility | 0.01 |
Model | Template_relevance |
Template Set | Pistachio/Bkms_metabolic/Pistachio_ringbreaker/Reaxys/Reaxys_biocatalysis |
Top-N result to add to graph | 6 |
Feasible Synthetic Routes
Q1: What are the primary molecular targets of haemanthamine?
A1: this compound interacts with various cellular targets. It has demonstrated inhibitory activity against both acetylcholinesterase (AChE) [, ] and butyrylcholinesterase (BuChE) []. Additionally, it exhibits potent inhibition of protein synthesis by binding to the ribosome and interfering with the polypeptide elongation stage [].
Q2: How does this compound’s inhibition of protein synthesis contribute to its anticancer activity?
A2: this compound’s inhibition of protein synthesis disproportionately affects rapidly dividing cells like cancer cells. By disrupting ribosome biogenesis, particularly the production of mature ribosomes, this compound limits the protein synthesis necessary for cancer cell growth and survival [].
Q3: How does this compound’s interaction with the actin cytoskeleton contribute to its anticancer activity?
A3: Research suggests that this compound can induce cytostatic effects in cancer cells, particularly glioblastoma cells, by rigidifying the actin cytoskeleton []. This disruption of the cytoskeleton can impair cell division, migration, and invasion, ultimately limiting tumor growth.
Q4: What is the molecular formula and weight of this compound?
A4: The molecular formula of this compound is C17H19NO4, and its molecular weight is 301.34 g/mol.
Q5: Which spectroscopic techniques are commonly employed to characterize this compound?
A5: Several spectroscopic methods are used to characterize this compound, including nuclear magnetic resonance (NMR) spectroscopy [, , , ], mass spectrometry (MS) [, , , , ], circular dichroism (CD) spectroscopy [, , , ], vibrational circular dichroism (VCD) [, ], and infrared (IR) spectroscopy []. These techniques provide valuable information about the compound's structure, stereochemistry, and purity.
Q6: What are the challenges associated with the stability and formulation of this compound?
A6: this compound exhibits chemical instability and poor water solubility, limiting its therapeutic applications []. These properties pose challenges in developing stable and effective formulations.
Q7: How can the stability and solubility of this compound be improved for pharmaceutical applications?
A7: Research suggests that incorporating this compound into amphiphilic electrospun nanofibers (NFs) can enhance its stability and solubility []. These NFs, composed of phosphatidylcholine (PC) and polyvinylpyrrolidone (PVP), act as templates for self-assembled liposomes, improving the drug's dissolution and bioavailability.
Q8: Does this compound exhibit any known catalytic properties?
A8: Currently, there is no research available suggesting that this compound possesses catalytic properties. The available literature focuses primarily on its biological activities and potential therapeutic applications.
Q9: How has computational chemistry been employed in this compound research?
A9: Computational techniques, such as molecular docking, have been employed to study the interactions between this compound and its molecular targets [, ]. These studies provide insights into the binding affinities and potential mechanisms of action of this compound and its derivatives. Additionally, quantitative structure-activity relationship (QSAR) models can be developed to predict the activity and properties of this compound analogues based on their structural features.
Q10: How do structural modifications of this compound influence its activity and selectivity?
A10: Research on this compound derivatives suggests that structural modifications, particularly at the C11 position, can significantly impact its biological activity and target selectivity [, ]. For instance, introducing specific benzoyl groups at the C11 hydroxyl group of ambelline, a closely related alkaloid, enhanced its inhibitory activity against the hepatic stage of Plasmodium infection while showing no activity against the blood stage []. In contrast, similar modifications on this compound did not yield significant activity against either stage, highlighting the importance of subtle structural changes in determining biological activity and selectivity.
Q11: What strategies have been explored to enhance this compound stability in formulations?
A11: Encapsulation of this compound in nanocarriers, such as liposomes formed from amphiphilic electrospun nanofibers [], has been investigated as a strategy to improve stability. These formulations protect the drug from degradation and can potentially enhance its solubility and bioavailability.
Q12: What is currently known about the pharmacokinetic profile of this compound?
A12: A pilot pharmacokinetic study in rats revealed that after intravenous administration of this compound, the compound was detected in plasma, bile, and urine []. This study provided preliminary data on the absorption, distribution, metabolism, and excretion (ADME) of this compound.
Q13: What in vitro models have been used to study the anticancer activity of this compound?
A13: The anticancer activity of this compound has been investigated in various in vitro models, including human colon cancer cell lines [] and glioblastoma cells []. These studies have demonstrated the ability of this compound to inhibit cell proliferation, adhesion, and invasion, as well as to modulate the expression of specific cytokines and matrix metalloproteinases.
Q14: Has the efficacy of this compound been evaluated in any in vivo models of disease?
A14: Yes, the in vivo efficacy of this compound has been evaluated in a zebrafish model of anxiety []. Alkaloid fractions from Griffinia species, including this compound, exhibited anxiolytic effects in the light-dark box test. Further investigation revealed that the anxiolytic action involved the GABAergic and serotonergic receptors.
Q15: What is the current understanding of the toxicity profile of this compound?
A15: While this compound exhibits promising biological activities, further research is needed to fully elucidate its toxicity profile. The available literature does not provide comprehensive data on long-term effects or potential adverse events.
Q16: What drug delivery systems have been explored for this compound?
A16: Electrospun nanofibers loaded with this compound, phosphatidylcholine, and polyvinylpyrrolidone have been explored as a potential drug delivery system []. This approach aims to enhance this compound's stability, solubility, and bioavailability for therapeutic applications.
Q17: Which analytical methods are used to quantify this compound in plant material and biological samples?
A17: Gas chromatography-mass spectrometry (GC-MS) is frequently employed for the identification and quantification of this compound and other Amaryllidaceae alkaloids in plant extracts [, , , , , , ]. High-performance liquid chromatography (HPLC) can also be used for separation and quantification, especially when coupled with sensitive detectors like mass spectrometry [].
Q18: Is there information available regarding the environmental impact and degradation of this compound?
A18: The research papers provided do not address the environmental impact or degradation pathways of this compound. Further studies are needed to assess its potential ecotoxicological effects and develop strategies for mitigating any negative environmental impacts.
Q19: What is the impact of this compound’s solubility on its bioavailability and efficacy?
A19: this compound’s poor water solubility poses a challenge for its bioavailability and therapeutic efficacy [, ]. The rate and extent of dissolution directly influence the drug's absorption and distribution within the body. Developing formulations that enhance this compound's dissolution rate is crucial for achieving optimal therapeutic outcomes.
Q20: Have the analytical methods used to quantify this compound been validated?
A20: While the provided research papers utilize established analytical techniques like GC-MS and HPLC, specific details regarding the validation of these methods for this compound quantification are not explicitly described. It is essential to note that rigorous method validation, including assessing accuracy, precision, specificity, linearity, range, limit of detection, limit of quantification, robustness, and system suitability, is crucial for ensuring reliable and reproducible analytical results.
Q21: What is the historical context of this compound research?
A21: this compound was first isolated from the Amaryllidaceae family, known for its rich alkaloid content []. Early research focused on its chemical structure and potential medicinal properties. Over time, studies have uncovered various biological activities of this compound, leading to renewed interest in its therapeutic potential.
Q22: Are there alternative compounds or classes with similar biological activities to this compound?
A22: Yes, several other Amaryllidaceae alkaloids, such as lycorine, tazettine, and galanthamine, exhibit overlapping biological activities with this compound [, , , , , ]. Additionally, synthetic compounds targeting similar pathways or mechanisms of action as this compound are also being explored.
Disclaimer and Information on In-Vitro Research Products
Please be aware that all articles and product information presented on BenchChem are intended solely for informational purposes. The products available for purchase on BenchChem are specifically designed for in-vitro studies, which are conducted outside of living organisms. In-vitro studies, derived from the Latin term "in glass," involve experiments performed in controlled laboratory settings using cells or tissues. It is important to note that these products are not categorized as medicines or drugs, and they have not received approval from the FDA for the prevention, treatment, or cure of any medical condition, ailment, or disease. We must emphasize that any form of bodily introduction of these products into humans or animals is strictly prohibited by law. It is essential to adhere to these guidelines to ensure compliance with legal and ethical standards in research and experimentation.