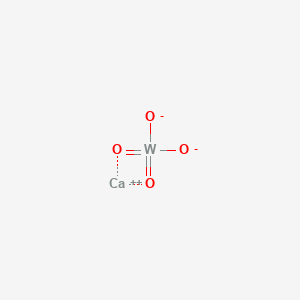
Calcium tungsten oxide (CaWO4)
Overview
Description
Calcium tungsten oxide, also known as calcium tungstate, is a chemical compound with the formula CaWO4. It is a white crystalline solid that occurs naturally as the mineral scheelite. This compound is significant due to its applications in various fields, including luminescence, photothermal therapy, and as a source of tungsten.
Mechanism of Action
Target of Action
Calcium tungsten oxide (CaWO4), also known as calcium;dioxido(dioxo)tungsten, is primarily targeted in optical applications due to its luminescent and scintillating properties . It is used as a laser host material for a variety of electronic applications . It is also used as a catalyst for the oxidation of organic substrates with hydrogen peroxide .
Mode of Action
When exposed to ionizing radiation, CaWO4 exhibits strong scintillation, converting the incident radiation into visible or ultraviolet photons . This conversion is a result of the interaction between the compound and its targets. In the presence of palladium (Pd), CaWO4 has been shown to catalyze the complete oxidation of different alkanes and toluene .
Biochemical Pathways
The biochemical pathways affected by CaWO4 are primarily related to its role as a catalyst in oxidation reactions . The compound facilitates the transformation of organic substrates through oxidation, leading to various downstream effects.
Result of Action
The primary result of CaWO4’s action is the emission of light, specifically visible or ultraviolet photons, when exposed to ionizing radiation . This property is leveraged in various applications, including radiation detection and medical imaging . When used as a catalyst, CaWO4 facilitates the oxidation of organic substrates .
Action Environment
The action of CaWO4 is influenced by environmental factors such as temperature and radiation exposure. The compound exhibits excellent thermal stability, making it well-suited for extreme conditions . Its luminescent properties are activated upon exposure to ionizing radiation . The stability and efficacy of CaWO4 as a catalyst or optical material can also be influenced by the presence of other substances, such as palladium in catalytic applications .
Preparation Methods
Synthetic Routes and Reaction Conditions: Calcium tungsten oxide can be synthesized through various methods. One common method involves the reaction of calcium chloride (CaCl2) with sodium tungstate (Na2WO4) in an aqueous solution. The reaction is typically carried out at room temperature, and the product is precipitated out of the solution: [ \text{CaCl}_2 + \text{Na}_2\text{WO}_4 \rightarrow \text{CaWO}_4 + 2\text{NaCl} ]
Industrial Production Methods: In industrial settings, calcium tungsten oxide is often produced by high-temperature solid-state reactions. For example, calcium carbonate (CaCO3) and tungsten trioxide (WO3) can be heated together at temperatures around 900-1000°C to produce calcium tungsten oxide: [ \text{CaCO}_3 + \text{WO}_3 \rightarrow \text{CaWO}_4 + \text{CO}_2 ]
Chemical Reactions Analysis
Types of Reactions: Calcium tungsten oxide undergoes various chemical reactions, including:
Oxidation and Reduction: It can participate in redox reactions, although it is generally stable under normal conditions.
Substitution Reactions: Calcium tungsten oxide can react with acids to form soluble tungstate salts and calcium salts.
Common Reagents and Conditions:
Acids: Reacts with hydrochloric acid (HCl) to form calcium chloride and tungstic acid. [ \text{CaWO}_4 + 2\text{HCl} \rightarrow \text{CaCl}_2 + \text{H}_2\text{WO}_4 ]
Major Products:
Calcium Chloride (CaCl2): Formed when reacting with hydrochloric acid.
Tungstic Acid (H2WO4): Formed as a result of acid reactions.
Scientific Research Applications
Calcium tungsten oxide has a wide range of applications in scientific research:
Luminescence: Used as a luminophore in various optical applications due to its ability to emit light when excited by ultraviolet radiation.
Photothermal Therapy: Utilized in cancer treatment for its ability to convert near-infrared light into heat, effectively destroying cancer cells.
Catalysis: Acts as a catalyst in various chemical reactions, including oxidation and reduction processes.
Gas Sensors: Employed in the development of gas sensors due to its sensitivity to changes in gas composition.
Comparison with Similar Compounds
Calcium tungsten oxide can be compared with other similar compounds such as:
Barium Tungsten Oxide (BaWO4): Similar in structure but has different luminescent properties.
Strontium Tungsten Oxide (SrWO4): Also similar in structure but used in different applications due to its unique properties.
Uniqueness: Calcium tungsten oxide is unique due to its combination of luminescent properties and its effectiveness in photothermal therapy. Its ability to convert near-infrared light into heat makes it particularly valuable in medical applications.
Properties
IUPAC Name |
calcium;dioxido(dioxo)tungsten | |
---|---|---|
Source | PubChem | |
URL | https://pubchem.ncbi.nlm.nih.gov | |
Description | Data deposited in or computed by PubChem | |
InChI |
InChI=1S/Ca.4O.W/q+2;;;2*-1; | |
Source | PubChem | |
URL | https://pubchem.ncbi.nlm.nih.gov | |
Description | Data deposited in or computed by PubChem | |
InChI Key |
FDMFQOCGNBYKPY-UHFFFAOYSA-N | |
Source | PubChem | |
URL | https://pubchem.ncbi.nlm.nih.gov | |
Description | Data deposited in or computed by PubChem | |
Canonical SMILES |
[O-][W](=O)(=O)[O-].[Ca+2] | |
Source | PubChem | |
URL | https://pubchem.ncbi.nlm.nih.gov | |
Description | Data deposited in or computed by PubChem | |
Molecular Formula |
CaO4W | |
Source | PubChem | |
URL | https://pubchem.ncbi.nlm.nih.gov | |
Description | Data deposited in or computed by PubChem | |
Molecular Weight |
287.92 g/mol | |
Source | PubChem | |
URL | https://pubchem.ncbi.nlm.nih.gov | |
Description | Data deposited in or computed by PubChem | |
Physical Description |
Dry Powder | |
Record name | Calcium tungsten oxide (CaWO4) | |
Source | EPA Chemicals under the TSCA | |
URL | https://www.epa.gov/chemicals-under-tsca | |
Description | EPA Chemicals under the Toxic Substances Control Act (TSCA) collection contains information on chemicals and their regulations under TSCA, including non-confidential content from the TSCA Chemical Substance Inventory and Chemical Data Reporting. | |
CAS No. |
7790-75-2 | |
Record name | Calcium tungstate(VI) | |
Source | ChemIDplus | |
URL | https://pubchem.ncbi.nlm.nih.gov/substance/?source=chemidplus&sourceid=0007790752 | |
Description | ChemIDplus is a free, web search system that provides access to the structure and nomenclature authority files used for the identification of chemical substances cited in National Library of Medicine (NLM) databases, including the TOXNET system. | |
Record name | Calcium tungsten oxide (CaWO4) | |
Source | EPA Chemicals under the TSCA | |
URL | https://www.epa.gov/chemicals-under-tsca | |
Description | EPA Chemicals under the Toxic Substances Control Act (TSCA) collection contains information on chemicals and their regulations under TSCA, including non-confidential content from the TSCA Chemical Substance Inventory and Chemical Data Reporting. | |
Synthesis routes and methods
Procedure details
Q1: What can be understood about the structural characteristics of Calcium tungsten oxide (CaWO4) nanoparticles synthesized using the solvothermal method described in the research?
A1: The research primarily focuses on synthesizing Calcium tungsten oxide (CaWO4) nanoparticles using a solvothermal method involving alkoxide precursors. While the study doesn't delve deep into definitive structural characterization of the final CaWO4 nanoparticles, it provides some critical insights:
- Initial Phase: The solvothermal reactions, employing various alcohols as modifiers, yielded powders initially identified as the scheelite phase of CaWO4 []. This identification was made using Transmission Electron Microscopy (TEM) and Powder X-ray Diffraction (PXRD).
- Need for Thermal Processing: The study highlights that these “initial” powders lacked luminescent properties, which are characteristic of CaWO4. This observation suggests that further thermal processing is crucial to achieve the desired crystallinity and optical properties in the synthesized nanoparticles [].
- Intermediate Identification: Interestingly, the research led to the identification of an intermediate compound, [(OEt)4W(μ-OEt)2Ca(DBP)2] (where DBP is 2,6-di-tert-butylphenolate) []. This finding offers valuable insight into the reaction pathway and potential control mechanisms for future synthesis optimization.
Disclaimer and Information on In-Vitro Research Products
Please be aware that all articles and product information presented on BenchChem are intended solely for informational purposes. The products available for purchase on BenchChem are specifically designed for in-vitro studies, which are conducted outside of living organisms. In-vitro studies, derived from the Latin term "in glass," involve experiments performed in controlled laboratory settings using cells or tissues. It is important to note that these products are not categorized as medicines or drugs, and they have not received approval from the FDA for the prevention, treatment, or cure of any medical condition, ailment, or disease. We must emphasize that any form of bodily introduction of these products into humans or animals is strictly prohibited by law. It is essential to adhere to these guidelines to ensure compliance with legal and ethical standards in research and experimentation.