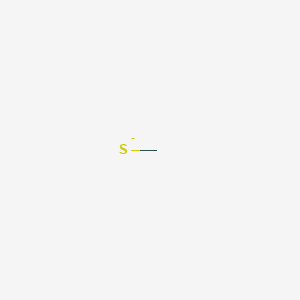
Methanethiolate
- Click on QUICK INQUIRY to receive a quote from our team of experts.
- With the quality product at a COMPETITIVE price, you can focus more on your research.
Overview
Description
Methanethiolate, also known as this compound, is an organosulfur compound with the chemical formula CH₃S⁻. It is the conjugate base of methanethiol (CH₃SH), which is a colorless gas with a distinctive putrid smell. This compound is a strong nucleophile and is commonly used in organic synthesis and various industrial applications .
Preparation Methods
Synthetic Routes and Reaction Conditions
Methanethiolate can be synthesized through the deprotonation of methanethiol using a strong base such as sodium hydroxide (NaOH) or potassium hydroxide (KOH). The reaction is typically carried out in an aqueous or alcoholic solution:
CH3SH+NaOH→CH3SNa+H2O
In industrial settings, methanethiol is often produced by the reaction of hydrogen sulfide (H₂S) with methanol (CH₃OH) in the presence of a catalyst. The resulting methanethiol can then be deprotonated to form mercaptomethanide .
Chemical Reactions Analysis
Types of Reactions
- Methanethiolate can be oxidized to form dimethyl disulfide (CH₃SSCH₃) or further to methanesulfonic acid (CH₃SO₃H).
Oxidation: 2CH3S−+[O]→CH3SSCH3+2e−
It can act as a reducing agent, particularly in the reduction of disulfides to thiols.Reduction: R−S−S−R+2CH3S−→2R−SH+CH3SSCH3
this compound is a strong nucleophile and can participate in nucleophilic substitution reactions with alkyl halides to form thioethers.Substitution: CH3S−+R−X→CH3SR+X−
Common Reagents and Conditions
Oxidizing agents: Hydrogen peroxide (H₂O₂), oxygen (O₂)
Reducing agents: Sodium borohydride (NaBH₄)
Solvents: Water, alcohols, and non-polar solvents like hexane
Major Products
- Dimethyl disulfide (CH₃SSCH₃)
- Methanesulfonic acid (CH₃SO₃H)
- Thioethers (R-S-R’)
Scientific Research Applications
Methanethiolate has a wide range of applications in scientific research:
- Chemistry : Used as a nucleophile in organic synthesis for the formation of carbon-sulfur bonds.
- Biology : Employed in the study of enzyme mechanisms, particularly those involving thiol groups.
- Medicine : Investigated for its potential in drug development, especially in the design of enzyme inhibitors.
- Industry : Utilized in the production of pesticides, pharmaceuticals, and as a corrosion inhibitor in the petroleum industry .
Mechanism of Action
Methanethiolate exerts its effects primarily through its strong nucleophilic properties. It can attack electrophilic centers in various molecules, leading to the formation of new chemical bonds. In biological systems, it can interact with thiol groups in proteins, affecting their structure and function. This interaction is crucial in the regulation of enzyme activity and cellular signaling pathways .
Comparison with Similar Compounds
Similar Compounds
- Ethanethiolate (C₂H₅S⁻)
- Propylthiolate (C₃H₇S⁻)
- Butylthiolate (C₄H₉S⁻)
Uniqueness
Methanethiolate is unique due to its small size and high nucleophilicity, making it highly reactive and versatile in various chemical reactions. Its simplicity and effectiveness in forming carbon-sulfur bonds distinguish it from other thiolates .
Properties
Molecular Formula |
CH3S- |
---|---|
Molecular Weight |
47.1 g/mol |
IUPAC Name |
methanethiolate |
InChI |
InChI=1S/CH4S/c1-2/h2H,1H3/p-1 |
InChI Key |
LSDPWZHWYPCBBB-UHFFFAOYSA-M |
Canonical SMILES |
C[S-] |
Synonyms |
methanethiol methanethiolate methylmercaptan methylmercaptan, lead (2+) salt methylmercaptan, mercury (2+) salt methylmercaptan, sodium salt |
Origin of Product |
United States |
Disclaimer and Information on In-Vitro Research Products
Please be aware that all articles and product information presented on BenchChem are intended solely for informational purposes. The products available for purchase on BenchChem are specifically designed for in-vitro studies, which are conducted outside of living organisms. In-vitro studies, derived from the Latin term "in glass," involve experiments performed in controlled laboratory settings using cells or tissues. It is important to note that these products are not categorized as medicines or drugs, and they have not received approval from the FDA for the prevention, treatment, or cure of any medical condition, ailment, or disease. We must emphasize that any form of bodily introduction of these products into humans or animals is strictly prohibited by law. It is essential to adhere to these guidelines to ensure compliance with legal and ethical standards in research and experimentation.