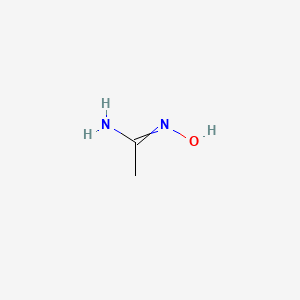
N-Hydroxyacetamidine
Overview
Description
N-Hydroxyacetamidine is an organic compound with the chemical formula C2H6N2O. It is a member of the amidoxime family, which are oximes where one of the substituents is an amino group. This compound is known for its role in various chemical reactions and its applications in scientific research, particularly in the fields of chemistry, biology, and medicine.
Preparation Methods
Synthetic Routes and Reaction Conditions
N-Hydroxyacetamidine can be synthesized through the reaction of acetonitrile with hydroxylamine. This reaction typically occurs under mild conditions and can be catalyzed by various bases such as calcium oxide. The reaction proceeds as follows: [ \text{CH}_3\text{CN} + \text{NH}_2\text{OH} \rightarrow \text{CH}_3\text{C}(NOH)\text{NH}_2 ]
Industrial Production Methods
In industrial settings, acetamidoxime is produced using similar methods but on a larger scale. The reaction conditions are optimized to ensure high yields and purity of the product. The use of continuous flow reactors and advanced purification techniques are common in industrial production.
Chemical Reactions Analysis
Types of Reactions
N-Hydroxyacetamidine undergoes various chemical reactions, including:
Oxidation: this compound can be oxidized to form nitriles or amides.
Reduction: It can be reduced to form primary amines.
Substitution: this compound can participate in nucleophilic substitution reactions.
Common Reagents and Conditions
Oxidation: Common oxidizing agents include hydrogen peroxide and potassium permanganate.
Reduction: Reducing agents such as lithium aluminum hydride or sodium borohydride are used.
Substitution: Nucleophiles such as halides or alkoxides can be used in substitution reactions.
Major Products Formed
Oxidation: Nitriles and amides.
Reduction: Primary amines.
Substitution: Various substituted amidoximes depending on the nucleophile used.
Scientific Research Applications
N-Hydroxyacetamidine has a wide range of applications in scientific research:
Chemistry: It is used as a ligand in coordination chemistry and as a precursor in the synthesis of various organic compounds.
Biology: this compound is studied for its potential as a nitric oxide donor, which has implications in cardiovascular research.
Medicine: It is explored for its potential therapeutic effects, including its use as a prodrug for the treatment of certain diseases.
Mechanism of Action
The mechanism of action of acetamidoxime involves its ability to donate nitric oxide (NO) upon oxidation. This process is catalyzed by enzymes such as cytochrome P450. The nitric oxide released can then interact with various molecular targets, including guanylate cyclase, leading to the relaxation of smooth muscle cells and vasodilation .
Comparison with Similar Compounds
Similar Compounds
Acetaldoxime: Similar in structure but lacks the amino group present in acetamidoxime.
Benzamidoxime: Contains a benzene ring instead of the methyl group found in acetamidoxime.
Uniqueness
N-Hydroxyacetamidine is unique due to its dual functionality as both an oxime and an amine. This allows it to participate in a wider range of chemical reactions compared to similar compounds. Additionally, its ability to act as a nitric oxide donor makes it particularly valuable in biomedical research .
Properties
Molecular Formula |
C2H6N2O |
---|---|
Molecular Weight |
74.08 g/mol |
IUPAC Name |
N'-hydroxyethanimidamide |
InChI |
InChI=1S/C2H6N2O/c1-2(3)4-5/h5H,1H3,(H2,3,4) |
InChI Key |
AEXITZJSLGALNH-UHFFFAOYSA-N |
Canonical SMILES |
CC(=NO)N |
Pictograms |
Irritant |
Synonyms |
acetohydroxamic acid Lithostat N-hydroxyacetamide N-hydroxyacetamidine Uronefrex |
Origin of Product |
United States |
Synthesis routes and methods
Procedure details
Disclaimer and Information on In-Vitro Research Products
Please be aware that all articles and product information presented on BenchChem are intended solely for informational purposes. The products available for purchase on BenchChem are specifically designed for in-vitro studies, which are conducted outside of living organisms. In-vitro studies, derived from the Latin term "in glass," involve experiments performed in controlled laboratory settings using cells or tissues. It is important to note that these products are not categorized as medicines or drugs, and they have not received approval from the FDA for the prevention, treatment, or cure of any medical condition, ailment, or disease. We must emphasize that any form of bodily introduction of these products into humans or animals is strictly prohibited by law. It is essential to adhere to these guidelines to ensure compliance with legal and ethical standards in research and experimentation.