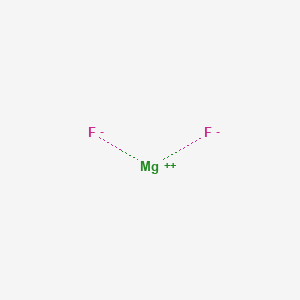
Magnesium fluoride
Overview
Description
Magnesium fluoride is a phthalazinone derivative known for its potent antihistamine properties. It primarily acts as a selective antagonist of histamine H1-receptors, which are involved in allergic reactions. This compound is used to alleviate symptoms associated with allergic rhinitis, such as sneezing, nasal congestion, and itching .
Preparation Methods
Synthetic Routes and Reaction Conditions: Magnesium fluoride is synthesized through a multi-step process involving the reaction of phthalazinone with various reagents. The synthesis typically involves the following steps:
Nitration: Phthalazinone is nitrated using a mixture of concentrated sulfuric acid and nitric acid to form nitrophthalazinone.
Reduction: The nitro group is reduced to an amino group using a reducing agent such as hydrogen gas in the presence of a palladium catalyst.
Substitution: The amino group is then substituted with a suitable alkyl or aryl group to form the final product, this compound.
Industrial Production Methods: Industrial production of this compound involves large-scale synthesis using automated reactors. The process is optimized for high yield and purity, with stringent control over reaction conditions such as temperature, pressure, and pH. The final product is purified using techniques like crystallization and chromatography .
Chemical Reactions Analysis
Types of Reactions: Magnesium fluoride undergoes several types of chemical reactions, including:
Oxidation: this compound can be oxidized to form various oxidized derivatives.
Reduction: Reduction of this compound can lead to the formation of reduced derivatives.
Substitution: this compound can undergo substitution reactions where functional groups are replaced with other groups.
Common Reagents and Conditions:
Oxidation: Common oxidizing agents include potassium permanganate and hydrogen peroxide.
Reduction: Reducing agents such as hydrogen gas with a palladium catalyst are used.
Substitution: Substitution reactions often involve reagents like alkyl halides or aryl halides under basic conditions.
Major Products:
Scientific Research Applications
Magnesium fluoride has a wide range of applications in scientific research:
Chemistry: this compound is used as a reagent in organic synthesis and as a standard in analytical chemistry.
Biology: It is used in studies related to histamine receptors and allergic reactions.
Medicine: this compound is used in the development of antihistamine drugs for treating allergic conditions.
Industry: It is used in the formulation of nasal sprays and eye drops for allergy relief.
Mechanism of Action
Magnesium fluoride exerts its effects by selectively binding to histamine H1-receptors, thereby blocking the action of histamine. This prevents the typical allergic symptoms such as itching, sneezing, and nasal congestion. The molecular targets include nerve endings, smooth muscle cells, and glandular cells. The pathways involved include the inhibition of histamine-mediated signaling, leading to reduced allergic responses .
Comparison with Similar Compounds
Cetirizine: Another antihistamine with similar H1-receptor antagonist properties.
Loratadine: A long-acting antihistamine used for allergy relief.
Fexofenadine: Known for its non-sedative properties and used in treating allergic rhinitis.
Uniqueness of Magnesium fluoride: this compound is unique due to its rapid onset of action and long duration of effect. It also has additional anti-inflammatory properties, making it effective in reducing the concentration of leukotrienes, kinins, and platelet-activating factors .
Properties
CAS No. |
7783-40-6 |
---|---|
Molecular Formula |
F2Mg |
Molecular Weight |
62.302 g/mol |
IUPAC Name |
magnesium;difluoride |
InChI |
InChI=1S/2FH.Mg/h2*1H;/q;;+2/p-2 |
InChI Key |
ORUIBWPALBXDOA-UHFFFAOYSA-L |
SMILES |
[F-].[F-].[Mg+2] |
Canonical SMILES |
[F-].[F-].[Mg+2] |
Key on ui other cas no. |
7783-40-6 |
physical_description |
OtherSolid; PelletsLargeCrystals |
Synonyms |
4-((4-chlorophenyl)methyl)-2- (hexahydro-1-methyl-1H-azepin-4-yl)-1(2H)- phthalazinone HCl 4-(p-chlorobenzyl)-2-(N-methylperhydroazepinyl-(4))-1-(2H)-phthalazinone A 5610 A-5610 Afluon Allergodil Astelin azelastine azelastine hydrochloride Azeptin Corifina Loxin Optilast Optivar Rhinolast Vividrin akut Azelastin |
Origin of Product |
United States |
Synthesis routes and methods
Procedure details
Q1: What is the mechanism of action of Azelastine Hydrochloride in treating allergic rhinitis?
A1: Azelastine Hydrochloride is a potent topical antihistamine with additional properties contributing to its efficacy in treating allergic rhinitis.
- H1-receptor antagonist: Azelastine primarily acts by blocking histamine binding to H1 receptors [, ]. This inhibits the cascade of inflammatory responses triggered by histamine release from mast cells upon exposure to allergens, such as sneezing, itching, and rhinorrhea.
- Mast cell stabilization: Azelastine also exhibits mast cell-stabilizing properties, further reducing the release of histamine and other inflammatory mediators [, ].
- Anti-inflammatory effects: Beyond histamine blockade, Azelastine has been shown to reduce the concentration of other inflammatory mediators like leukotrienes, kinins, and platelet-activating factor, both in vitro and in vivo []. This contributes to a broader anti-inflammatory effect in the nasal mucosa.
Q2: What is the evidence for the efficacy of Azelastine Hydrochloride nasal spray in treating allergic rhinitis?
A2: Clinical studies have demonstrated the efficacy and safety of Azelastine Hydrochloride nasal spray in treating both seasonal and perennial allergic rhinitis in adults and children [, ].
- Symptom relief: Studies show significant reductions in all cardinal symptoms of allergic rhinitis, including sneezing, nasal itching, congestion, rhinorrhea, and ocular symptoms like itching and tearing [, ].
- Rapid onset of action: One of the key advantages of Azelastine nasal spray is its rapid onset of action, providing relief within minutes of administration [, ].
- Efficacy compared to other treatments: Azelastine has shown comparable or superior efficacy to other intranasal corticosteroids, with a faster onset of action and a favorable safety profile [].
Q3: Besides its pharmaceutical applications, is Azelastine Hydrochloride used in other scientific research areas?
A3: While the provided research focuses on Azelastine Hydrochloride's role in treating allergic rhinitis, its chemical properties are also relevant to other research areas:
- Fluohydric acid reclamation: Interestingly, one study [] describes a method for reclaiming Fluohydric acid from industrial wastewater using a process involving Azelastine Hydrochloride (referred to as "Afluon (Asta)" in the paper). This highlights the compound's potential application beyond its pharmaceutical use.
Disclaimer and Information on In-Vitro Research Products
Please be aware that all articles and product information presented on BenchChem are intended solely for informational purposes. The products available for purchase on BenchChem are specifically designed for in-vitro studies, which are conducted outside of living organisms. In-vitro studies, derived from the Latin term "in glass," involve experiments performed in controlled laboratory settings using cells or tissues. It is important to note that these products are not categorized as medicines or drugs, and they have not received approval from the FDA for the prevention, treatment, or cure of any medical condition, ailment, or disease. We must emphasize that any form of bodily introduction of these products into humans or animals is strictly prohibited by law. It is essential to adhere to these guidelines to ensure compliance with legal and ethical standards in research and experimentation.