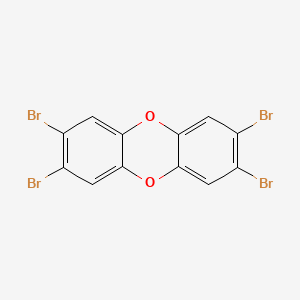
2,3,7,8-Tetrabromodibenzo-P-dioxin
Overview
Description
2,3,7,8-Tetrabromodibenzo-P-dioxin is an organobromine compound that belongs to the family of polybrominated dibenzo-p-dioxins. It is characterized by the presence of four bromine atoms at positions 2, 3, 7, and 8 on the dibenzo-p-dioxin structure. This compound is known for its environmental persistence and potential toxicological effects .
Mechanism of Action
Target of Action
The primary target of 2,3,7,8-Tetrabromodibenzo-P-dioxin (TBDD) is the aryl hydrocarbon receptor (AhR) . AhR is a transcription factor present in all cells and is involved in the expression of several genes . It plays a crucial role in the regulation of biological responses to planar aromatic hydrocarbons .
Mode of Action
TBDD interacts with its target, the AhR, by binding to it . This binding induces the production of endogenous ligands such as aspartate aminotransferase, indolethylamine N-methyltransferase, and aldehyde dehydrogenases . The interaction of TBDD with AhR leads to changes in the expression of several hundred genes .
Biochemical Pathways
The binding of TBDD to AhR affects various biochemical pathways. It induces the production of enzymes responsible for the breakdown of foreign and often toxic compounds, a process known as enzyme induction . It also prompts the production of proteins that reduce oxidative stress, such as superoxide dismutase and peroxiredoxins .
Pharmacokinetics
TBDD exhibits lipophilic properties, which allows it to easily undergo bioaccumulation . The elimination half-life of TBDD from the liver is estimated to range from 12 to 16 days .
Result of Action
The molecular and cellular effects of TBDD’s action are diverse. It can induce lymphocyte apoptosis, inhibit the production of cytotoxic T cells, and promote the high expression of immune suppression factors . This leads to immune system regulatory dysfunction . TBDD can also invade DNA molecules, inducing mutations, and thereby having teratogenic and carcinogenic effects .
Action Environment
Environmental factors can influence the action, efficacy, and stability of TBDD. Its high stability allows it to persist in the environment for a long time . It can form aerosols, making it easy to spread . The toxicity of TBDD can vary among different species, indicating that the biological environment can also influence its action .
Biochemical Analysis
Biochemical Properties
2,3,7,8-Tetrabromodibenzo-P-dioxin plays a significant role in biochemical reactions, particularly through its interactions with various enzymes, proteins, and other biomolecules. One of the primary interactions is with the aryl hydrocarbon receptor (AhR), a transcription factor that regulates the expression of genes involved in xenobiotic metabolism. Upon binding to AhR, this compound activates the receptor, leading to the induction of phase I and phase II detoxifying enzymes such as cytochrome P450 1A1 (CYP1A1) and glutathione S-transferase (GST). These interactions facilitate the metabolism and detoxification of the compound, although they may also contribute to its toxic effects .
Cellular Effects
This compound exerts various effects on different cell types and cellular processes. It influences cell function by modulating cell signaling pathways, gene expression, and cellular metabolism. For instance, the compound has been shown to affect the expression of genes involved in cell cycle regulation, apoptosis, and immune responses. In hepatocytes, this compound induces the expression of enzymes involved in lipid metabolism, leading to hepatic steatosis and lipid accumulation . Additionally, it can suppress the differentiation and function of B-cells, thereby impairing humoral immune responses .
Molecular Mechanism
The molecular mechanism of action of this compound involves its binding to the aryl hydrocarbon receptor (AhR) Upon binding, the AhR translocates to the nucleus, where it dimerizes with the AhR nuclear translocator (ARNT)These genes encode for enzymes such as cytochrome P450 1A1 (CYP1A1) and glutathione S-transferase (GST), which are involved in the metabolism and detoxification of xenobiotics . Additionally, this compound can induce oxidative stress and disrupt cellular redox balance, contributing to its toxic effects .
Temporal Effects in Laboratory Settings
In laboratory settings, the effects of this compound can change over time. The compound is relatively stable and resistant to degradation, leading to its persistence in biological systems. Studies have shown that the effects of this compound on cellular function can be observed over extended periods. For example, in rat liver, the compound induces persistent increases in the activities of hepatic microsomal enzymes and serum lipid levels . The elimination half-life of this compound from the liver is estimated to range from 12 to 16 days .
Dosage Effects in Animal Models
The effects of this compound vary with different dosages in animal models. At lower doses, the compound induces mild biochemical changes, such as increased enzyme activities and lipid accumulation. At higher doses, it can cause severe toxic effects, including hepatotoxicity, thymic atrophy, and myelotoxicity . In rats, doses of 30 μg/kg and higher have been associated with significant adverse effects, including growth retardation, decreased bone marrow hematopoiesis, and increased mortality .
Metabolic Pathways
This compound is involved in various metabolic pathways, primarily through its interactions with enzymes such as cytochrome P450 1A1 (CYP1A1) and glutathione S-transferase (GST). These enzymes facilitate the metabolism and detoxification of the compound by catalyzing its oxidation and conjugation reactions . The compound can also induce lipid metabolism disorders by affecting the ROS/AMPK/CD36 signaling pathway, leading to hepatic steatosis and lipid accumulation .
Transport and Distribution
The transport and distribution of this compound within cells and tissues are influenced by its interactions with transporters and binding proteins. The compound is highly lipophilic, allowing it to accumulate in adipose tissue and other lipid-rich compartments . In the liver, this compound is sequestered by the cytochrome P450 enzyme CYP1A2, which facilitates its retention and metabolism . The distribution of the compound is dose-dependent, with higher doses leading to increased sequestration in the liver and adipose tissue .
Subcellular Localization
The subcellular localization of this compound is primarily determined by its interactions with the aryl hydrocarbon receptor (AhR). Upon binding to AhR, the compound is translocated to the nucleus, where it exerts its effects on gene expression . Additionally, this compound can localize to the endoplasmic reticulum, where it interacts with cytochrome P450 enzymes involved in its metabolism . The compound’s lipophilic nature also allows it to accumulate in lipid droplets and other lipid-rich organelles .
Preparation Methods
Synthetic Routes and Reaction Conditions: The synthesis of 2,3,7,8-Tetrabromodibenzo-P-dioxin typically involves the bromination of dibenzo-p-dioxin. The reaction is carried out using bromine or bromine-containing reagents under controlled conditions to ensure selective bromination at the desired positions .
Industrial Production Methods: Industrial production of this compound is not common due to its toxicological properties. it can be produced as a byproduct during the manufacture of brominated flame retardants and other brominated organic compounds .
Chemical Reactions Analysis
Types of Reactions: 2,3,7,8-Tetrabromodibenzo-P-dioxin can undergo various chemical reactions, including:
Oxidation: The compound can be oxidized under specific conditions to form brominated quinones.
Reduction: Reduction reactions can lead to the formation of less brominated dibenzo-p-dioxins.
Substitution: Halogen exchange reactions can occur, where bromine atoms are replaced by other halogens.
Common Reagents and Conditions:
Oxidation: Strong oxidizing agents such as potassium permanganate or chromium trioxide.
Reduction: Reducing agents like sodium borohydride or lithium aluminum hydride.
Substitution: Halogen exchange can be facilitated by reagents like sodium iodide in acetone.
Major Products:
Oxidation: Brominated quinones.
Reduction: Less brominated dibenzo-p-dioxins.
Substitution: Dibenzodioxins with different halogen atoms.
Scientific Research Applications
2,3,7,8-Tetrabromodibenzo-P-dioxin has been studied extensively for its environmental and toxicological impacts. Its applications in scientific research include:
Environmental Chemistry: Used as a model compound to study the behavior and fate of brominated dioxins in the environment.
Toxicology: Investigated for its toxic effects on various biological systems, including its potential to cause cancer and disrupt endocrine functions.
Analytical Chemistry: Employed in the development of analytical methods for detecting and quantifying brominated dioxins in environmental samples
Comparison with Similar Compounds
2,3,7,8-Tetrachlorodibenzo-P-dioxin: A closely related compound with chlorine atoms instead of bromine. It is known for its high toxicity and environmental persistence.
Polybrominated Dibenzofurans: Compounds with similar structures but different halogenation patterns.
Uniqueness: 2,3,7,8-Tetrabromodibenzo-P-dioxin is unique due to its specific bromination pattern, which influences its chemical reactivity and toxicological properties. Compared to its chlorinated counterpart, it may exhibit different environmental behaviors and biological effects .
Properties
IUPAC Name |
2,3,7,8-tetrabromodibenzo-p-dioxin | |
---|---|---|
Source | PubChem | |
URL | https://pubchem.ncbi.nlm.nih.gov | |
Description | Data deposited in or computed by PubChem | |
InChI |
InChI=1S/C12H4Br4O2/c13-5-1-9-10(2-6(5)14)18-12-4-8(16)7(15)3-11(12)17-9/h1-4H | |
Source | PubChem | |
URL | https://pubchem.ncbi.nlm.nih.gov | |
Description | Data deposited in or computed by PubChem | |
InChI Key |
JZLQUWSWOJPCAK-UHFFFAOYSA-N | |
Source | PubChem | |
URL | https://pubchem.ncbi.nlm.nih.gov | |
Description | Data deposited in or computed by PubChem | |
Canonical SMILES |
C1=C2C(=CC(=C1Br)Br)OC3=CC(=C(C=C3O2)Br)Br | |
Source | PubChem | |
URL | https://pubchem.ncbi.nlm.nih.gov | |
Description | Data deposited in or computed by PubChem | |
Molecular Formula |
C12H4Br4O2 | |
Source | PubChem | |
URL | https://pubchem.ncbi.nlm.nih.gov | |
Description | Data deposited in or computed by PubChem | |
DSSTOX Substance ID |
DTXSID6073524 | |
Record name | 2,3,7,8-Tetrabromodibenzo-p-dioxin | |
Source | EPA DSSTox | |
URL | https://comptox.epa.gov/dashboard/DTXSID6073524 | |
Description | DSSTox provides a high quality public chemistry resource for supporting improved predictive toxicology. | |
Molecular Weight |
499.77 g/mol | |
Source | PubChem | |
URL | https://pubchem.ncbi.nlm.nih.gov | |
Description | Data deposited in or computed by PubChem | |
CAS No. |
50585-41-6 | |
Record name | 2,3,7,8-Tetrabromodibenzo-p-dioxin | |
Source | CAS Common Chemistry | |
URL | https://commonchemistry.cas.org/detail?cas_rn=50585-41-6 | |
Description | CAS Common Chemistry is an open community resource for accessing chemical information. Nearly 500,000 chemical substances from CAS REGISTRY cover areas of community interest, including common and frequently regulated chemicals, and those relevant to high school and undergraduate chemistry classes. This chemical information, curated by our expert scientists, is provided in alignment with our mission as a division of the American Chemical Society. | |
Explanation | The data from CAS Common Chemistry is provided under a CC-BY-NC 4.0 license, unless otherwise stated. | |
Record name | 2,3,7,8-Tetrabromodibenzo-4-dioxin | |
Source | ChemIDplus | |
URL | https://pubchem.ncbi.nlm.nih.gov/substance/?source=chemidplus&sourceid=0050585416 | |
Description | ChemIDplus is a free, web search system that provides access to the structure and nomenclature authority files used for the identification of chemical substances cited in National Library of Medicine (NLM) databases, including the TOXNET system. | |
Record name | 2,3,7,8-Tetrabromodibenzo-p-dioxin | |
Source | EPA DSSTox | |
URL | https://comptox.epa.gov/dashboard/DTXSID6073524 | |
Description | DSSTox provides a high quality public chemistry resource for supporting improved predictive toxicology. | |
Record name | 2,3,7,8-TETRABROMODIBENZO-P-DIOXIN | |
Source | FDA Global Substance Registration System (GSRS) | |
URL | https://gsrs.ncats.nih.gov/ginas/app/beta/substances/04PL7F455E | |
Description | The FDA Global Substance Registration System (GSRS) enables the efficient and accurate exchange of information on what substances are in regulated products. Instead of relying on names, which vary across regulatory domains, countries, and regions, the GSRS knowledge base makes it possible for substances to be defined by standardized, scientific descriptions. | |
Explanation | Unless otherwise noted, the contents of the FDA website (www.fda.gov), both text and graphics, are not copyrighted. They are in the public domain and may be republished, reprinted and otherwise used freely by anyone without the need to obtain permission from FDA. Credit to the U.S. Food and Drug Administration as the source is appreciated but not required. | |
Retrosynthesis Analysis
AI-Powered Synthesis Planning: Our tool employs the Template_relevance Pistachio, Template_relevance Bkms_metabolic, Template_relevance Pistachio_ringbreaker, Template_relevance Reaxys, Template_relevance Reaxys_biocatalysis model, leveraging a vast database of chemical reactions to predict feasible synthetic routes.
One-Step Synthesis Focus: Specifically designed for one-step synthesis, it provides concise and direct routes for your target compounds, streamlining the synthesis process.
Accurate Predictions: Utilizing the extensive PISTACHIO, BKMS_METABOLIC, PISTACHIO_RINGBREAKER, REAXYS, REAXYS_BIOCATALYSIS database, our tool offers high-accuracy predictions, reflecting the latest in chemical research and data.
Strategy Settings
Precursor scoring | Relevance Heuristic |
---|---|
Min. plausibility | 0.01 |
Model | Template_relevance |
Template Set | Pistachio/Bkms_metabolic/Pistachio_ringbreaker/Reaxys/Reaxys_biocatalysis |
Top-N result to add to graph | 6 |
Feasible Synthetic Routes
A: 2,3,7,8-TBDD, similar to TCDD, exerts its effects by binding to the aryl hydrocarbon receptor (AhR) [, , , , , ]. This binding triggers a cascade of events, including:
- AhR Activation & Nuclear Translocation: 2,3,7,8-TBDD binds to the cytosolic AhR, causing its activation and translocation to the nucleus [, , , ].
- Gene Transcription Modulation: The activated AhR complex binds to specific DNA sequences, influencing the transcription of genes involved in xenobiotic metabolism, such as CYP1A1, CYP1A2, and glutathione S-transferase [, , , ].
- Enzyme Induction: Increased expression of these genes leads to elevated levels of enzymes like aryl hydrocarbon hydroxylase (AHH), ethoxyresorufin-O-deethylase (EROD), and ethoxycoumarin O-deethylase (ECOD) in the liver, lung, and skin [, , ].
- Toxicological Effects: While the exact mechanisms of toxicity are not fully elucidated, enzyme induction, altered gene expression, and potential oxidative stress are implicated in the observed toxic effects of 2,3,7,8-TBDD [, , , , , ].
ANone: 2,3,7,8-TBDD possesses the following structural characteristics:
- Spectroscopic Data: Detailed spectroscopic information can be found in the research focusing on its synthesis and analytical methods [].
A: While specific information on material compatibility is limited within the provided research, 2,3,7,8-TBDD, like many halogenated aromatic hydrocarbons, is known to be persistent and resistant to degradation in the environment [, ]. This stability raises concerns about its potential for bioaccumulation and long-term environmental impact.
ANone: The research provided focuses primarily on the toxicological and environmental aspects of 2,3,7,8-TBDD. There is no indication of any catalytic properties or applications for this compound.
A: Yes, computational approaches have been used to estimate the octanol-water partition coefficient (Kow) of 2,3,7,8-TBDD [, ]. This parameter is crucial for understanding the compound's lipophilicity and predicting its distribution and potential for bioaccumulation.
A: SAR studies highlight the importance of halogen substitution in the dioxin structure [, , , ].
- Halogen Type: The type of halogen influences potency, with chlorinated dioxins generally considered more potent than brominated ones [, , ].
- Halogen Position: The position of halogens on the dibenzo-p-dioxin ring system significantly impacts AhR binding affinity and toxicity, with 2,3,7,8-substitution being the most potent configuration [, , ].
- Congener-Specific Effects: Research shows that even slight structural variations among polyhalogenated dibenzo-p-dioxins, dibenzofurans, and biphenyls can lead to differences in absorption, distribution, metabolism, and excretion (ADME) profiles, ultimately affecting their toxicity [, , ].
A: 2,3,7,8-TBDD demonstrates significant stability in various environmental matrices [, ], making it a persistent environmental contaminant. The provided research primarily focuses on its toxicological assessment, and details regarding specific formulations aimed at improving stability, solubility, or bioavailability are not discussed.
A: While specific regulations for 2,3,7,8-TBDD might vary, its structural similarity to TCDD, classified as a persistent organic pollutant (POP) by the Stockholm Convention, suggests that similar stringent regulatory controls might be applicable or under consideration [, ].
Disclaimer and Information on In-Vitro Research Products
Please be aware that all articles and product information presented on BenchChem are intended solely for informational purposes. The products available for purchase on BenchChem are specifically designed for in-vitro studies, which are conducted outside of living organisms. In-vitro studies, derived from the Latin term "in glass," involve experiments performed in controlled laboratory settings using cells or tissues. It is important to note that these products are not categorized as medicines or drugs, and they have not received approval from the FDA for the prevention, treatment, or cure of any medical condition, ailment, or disease. We must emphasize that any form of bodily introduction of these products into humans or animals is strictly prohibited by law. It is essential to adhere to these guidelines to ensure compliance with legal and ethical standards in research and experimentation.