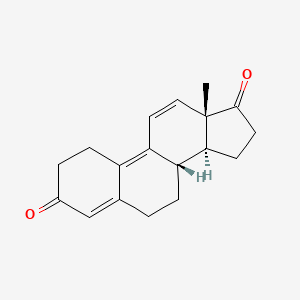
Trendione
Overview
Description
. It is structurally similar to trenbolone and functions as a precursor to it. This compound has been used in various applications, including as a performance-enhancing drug and in scientific research.
Preparation Methods
Synthetic Routes and Reaction Conditions
The synthesis of estra-4,9,11-triene-3,17-dione typically involves the use of dioscin as a raw material. The dioscin undergoes pressure cracking, oxidation, and hydrolysis at 200°C to obtain ketene acetate. This ketene acetate is then subjected to Beckmann rearrangement to introduce a carbonyl group on the 17th carbon .
Industrial Production Methods
Industrial production methods for estra-4,9,11-triene-3,17-dione are not widely documented. the general approach involves large-scale synthesis using similar reaction conditions as those used in laboratory settings, with appropriate scaling up of reagents and reaction vessels.
Chemical Reactions Analysis
Types of Reactions
Estra-4,9,11-triene-3,17-dione undergoes various chemical reactions, including:
Oxidation: Conversion to trenbolone.
Reduction: Formation of dihydro derivatives.
Substitution: Introduction of different functional groups at specific positions on the steroid backbone.
Common Reagents and Conditions
Common reagents used in these reactions include oxidizing agents like potassium permanganate and reducing agents like lithium aluminum hydride. Reaction conditions typically involve controlled temperatures and inert atmospheres to prevent unwanted side reactions.
Major Products Formed
The major products formed from these reactions include trenbolone and its derivatives, which are potent anabolic steroids with significant muscle-building properties .
Scientific Research Applications
Estra-4,9,11-triene-3,17-dione has several scientific research applications:
Chemistry: Used as a precursor in the synthesis of trenbolone and other anabolic steroids.
Biology: Studied for its effects on muscle growth and development.
Medicine: Investigated for potential therapeutic uses in conditions requiring anabolic steroids.
Mechanism of Action
Estra-4,9,11-triene-3,17-dione exerts its effects by converting to trenbolone in the body. Trenbolone binds to androgen receptors, leading to increased protein synthesis and muscle growth. The molecular targets include androgen receptors in muscle tissue, which mediate the anabolic effects .
Comparison with Similar Compounds
Similar Compounds
Trenbolone: A potent anabolic steroid with similar structure and effects.
Dienedione: Another synthetic anabolic steroid with similar properties but different metabolic pathways.
Uniqueness
Estra-4,9,11-triene-3,17-dione is unique in its role as a prohormone to trenbolone, providing a precursor that can be converted to the active steroid in the body. This makes it a valuable compound for research and potential therapeutic applications.
Properties
IUPAC Name |
(8S,13S,14S)-13-methyl-1,2,6,7,8,14,15,16-octahydrocyclopenta[a]phenanthrene-3,17-dione | |
---|---|---|
Source | PubChem | |
URL | https://pubchem.ncbi.nlm.nih.gov | |
Description | Data deposited in or computed by PubChem | |
InChI |
InChI=1S/C18H20O2/c1-18-9-8-14-13-5-3-12(19)10-11(13)2-4-15(14)16(18)6-7-17(18)20/h8-10,15-16H,2-7H2,1H3/t15-,16+,18+/m1/s1 | |
Source | PubChem | |
URL | https://pubchem.ncbi.nlm.nih.gov | |
Description | Data deposited in or computed by PubChem | |
InChI Key |
KBSXJBBFQODDTQ-RYRKJORJSA-N | |
Source | PubChem | |
URL | https://pubchem.ncbi.nlm.nih.gov | |
Description | Data deposited in or computed by PubChem | |
Canonical SMILES |
CC12C=CC3=C4CCC(=O)C=C4CCC3C1CCC2=O | |
Source | PubChem | |
URL | https://pubchem.ncbi.nlm.nih.gov | |
Description | Data deposited in or computed by PubChem | |
Isomeric SMILES |
C[C@]12C=CC3=C4CCC(=O)C=C4CC[C@H]3[C@@H]1CCC2=O | |
Source | PubChem | |
URL | https://pubchem.ncbi.nlm.nih.gov | |
Description | Data deposited in or computed by PubChem | |
Molecular Formula |
C18H20O2 | |
Source | PubChem | |
URL | https://pubchem.ncbi.nlm.nih.gov | |
Description | Data deposited in or computed by PubChem | |
DSSTOX Substance ID |
DTXSID80873491 | |
Record name | Estra-4,9,11-triene-3,17-dione | |
Source | EPA DSSTox | |
URL | https://comptox.epa.gov/dashboard/DTXSID80873491 | |
Description | DSSTox provides a high quality public chemistry resource for supporting improved predictive toxicology. | |
Molecular Weight |
268.3 g/mol | |
Source | PubChem | |
URL | https://pubchem.ncbi.nlm.nih.gov | |
Description | Data deposited in or computed by PubChem | |
CAS No. |
4642-95-9 | |
Record name | Trendione | |
Source | CAS Common Chemistry | |
URL | https://commonchemistry.cas.org/detail?cas_rn=4642-95-9 | |
Description | CAS Common Chemistry is an open community resource for accessing chemical information. Nearly 500,000 chemical substances from CAS REGISTRY cover areas of community interest, including common and frequently regulated chemicals, and those relevant to high school and undergraduate chemistry classes. This chemical information, curated by our expert scientists, is provided in alignment with our mission as a division of the American Chemical Society. | |
Explanation | The data from CAS Common Chemistry is provided under a CC-BY-NC 4.0 license, unless otherwise stated. | |
Record name | Trendione | |
Source | ChemIDplus | |
URL | https://pubchem.ncbi.nlm.nih.gov/substance/?source=chemidplus&sourceid=0004642959 | |
Description | ChemIDplus is a free, web search system that provides access to the structure and nomenclature authority files used for the identification of chemical substances cited in National Library of Medicine (NLM) databases, including the TOXNET system. | |
Record name | Estra-4,9,11-triene-3,17-dione | |
Source | EPA DSSTox | |
URL | https://comptox.epa.gov/dashboard/DTXSID80873491 | |
Description | DSSTox provides a high quality public chemistry resource for supporting improved predictive toxicology. | |
Record name | TRENDIONE | |
Source | FDA Global Substance Registration System (GSRS) | |
URL | https://gsrs.ncats.nih.gov/ginas/app/beta/substances/7M9J4CV848 | |
Description | The FDA Global Substance Registration System (GSRS) enables the efficient and accurate exchange of information on what substances are in regulated products. Instead of relying on names, which vary across regulatory domains, countries, and regions, the GSRS knowledge base makes it possible for substances to be defined by standardized, scientific descriptions. | |
Explanation | Unless otherwise noted, the contents of the FDA website (www.fda.gov), both text and graphics, are not copyrighted. They are in the public domain and may be republished, reprinted and otherwise used freely by anyone without the need to obtain permission from FDA. Credit to the U.S. Food and Drug Administration as the source is appreciated but not required. | |
Retrosynthesis Analysis
AI-Powered Synthesis Planning: Our tool employs the Template_relevance Pistachio, Template_relevance Bkms_metabolic, Template_relevance Pistachio_ringbreaker, Template_relevance Reaxys, Template_relevance Reaxys_biocatalysis model, leveraging a vast database of chemical reactions to predict feasible synthetic routes.
One-Step Synthesis Focus: Specifically designed for one-step synthesis, it provides concise and direct routes for your target compounds, streamlining the synthesis process.
Accurate Predictions: Utilizing the extensive PISTACHIO, BKMS_METABOLIC, PISTACHIO_RINGBREAKER, REAXYS, REAXYS_BIOCATALYSIS database, our tool offers high-accuracy predictions, reflecting the latest in chemical research and data.
Strategy Settings
Precursor scoring | Relevance Heuristic |
---|---|
Min. plausibility | 0.01 |
Model | Template_relevance |
Template Set | Pistachio/Bkms_metabolic/Pistachio_ringbreaker/Reaxys/Reaxys_biocatalysis |
Top-N result to add to graph | 6 |
Feasible Synthetic Routes
Disclaimer and Information on In-Vitro Research Products
Please be aware that all articles and product information presented on BenchChem are intended solely for informational purposes. The products available for purchase on BenchChem are specifically designed for in-vitro studies, which are conducted outside of living organisms. In-vitro studies, derived from the Latin term "in glass," involve experiments performed in controlled laboratory settings using cells or tissues. It is important to note that these products are not categorized as medicines or drugs, and they have not received approval from the FDA for the prevention, treatment, or cure of any medical condition, ailment, or disease. We must emphasize that any form of bodily introduction of these products into humans or animals is strictly prohibited by law. It is essential to adhere to these guidelines to ensure compliance with legal and ethical standards in research and experimentation.