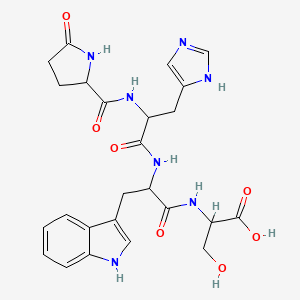
H-DL-Pyr-DL-His-DL-Trp-DL-Ser-OH
Overview
Description
H-DL-Pyr-DL-His-DL-Trp-DL-Ser-OH is a tetrapeptide composed of DL-configured amino acids: pyroglutamic acid (Pyr), histidine (His), tryptophan (Trp), and serine (Ser). This sequence combines aromatic (Trp), imidazole (His), and polar (Ser) side chains, along with a cyclic pyroglutamate moiety, which may influence its conformational stability and biochemical interactions.
Preparation Methods
Synthetic Routes and Reaction Conditions
The synthesis of H-DL-Pyr-DL-His-DL-Trp-DL-Ser-OH typically involves solid-phase peptide synthesis (SPPS). This method allows for the sequential addition of amino acids to a growing peptide chain anchored to a solid resin. The process involves the following steps:
Resin Loading: The first amino acid, pyrrolysine, is attached to the resin.
Deprotection: The protecting group on the amino acid is removed to allow for the next amino acid to be added.
Coupling: The next amino acid, histidine, is coupled to the growing peptide chain using a coupling reagent such as N,N’-diisopropylcarbodiimide (DIC) and 1-hydroxybenzotriazole (HOBt).
Repetition: Steps 2 and 3 are repeated for tryptophan and serine.
Cleavage: The completed peptide is cleaved from the resin using a cleavage reagent such as trifluoroacetic acid (TFA).
Industrial Production Methods
Industrial production of this compound follows similar principles but on a larger scale. Automated peptide synthesizers are often used to increase efficiency and yield. The process is optimized to ensure high purity and consistency of the final product.
Chemical Reactions Analysis
Types of Reactions
H-DL-Pyr-DL-His-DL-Trp-DL-Ser-OH can undergo various chemical reactions, including:
Oxidation: The tryptophan residue can be oxidized to form kynurenine.
Reduction: Disulfide bonds, if present, can be reduced to free thiols.
Substitution: Amino acid residues can be substituted with other functional groups to modify the peptide’s properties.
Common Reagents and Conditions
Oxidation: Hydrogen peroxide (H2O2) or performic acid can be used under mild conditions.
Reduction: Dithiothreitol (DTT) or tris(2-carboxyethyl)phosphine (TCEP) are common reducing agents.
Substitution: Various reagents, such as N-hydroxysuccinimide (NHS) esters, can be used for functional group substitution.
Major Products
Oxidation: Kynurenine and other oxidized derivatives.
Reduction: Free thiols from disulfide bond reduction.
Substitution: Modified peptides with altered functional groups.
Scientific Research Applications
The compound exhibits various biological activities due to its constituent amino acids, which include pyrrolidine (Pyr), histidine (His), tryptophan (Trp), and serine (Ser). Research indicates that peptides containing these amino acids can influence neurotransmission, hormone regulation, and immune responses. Specifically, compounds with tryptophan and tyrosine are noted for their roles in neurotransmission and hormone regulation.
Potential Therapeutic Applications:
- Neuroprotective Effects: The presence of tryptophan is associated with serotonin production, which can have neuroprotective effects.
- Anti-inflammatory Properties: Peptides like H-DL-Pyr-DL-His-DL-Trp-DL-Ser-OH have shown potential in reducing inflammation, making them candidates for treating inflammatory diseases.
- Analgesic Effects: Some studies suggest that similar peptides can exhibit analgesic properties, offering potential for pain management therapies.
Molecular Interaction Studies
Understanding how this compound interacts with biological receptors and enzymes is crucial for elucidating its mechanisms of action. Various techniques such as molecular docking studies are employed to investigate these interactions, revealing insights into how the compound exerts its biological effects .
Key Findings from Interaction Studies:
- The compound's structure allows it to bind effectively with specific receptors involved in neurotransmission and immune response modulation.
- Molecular docking studies have indicated strong binding affinities with targets relevant to neuropharmacology, suggesting its potential as a therapeutic agent in neurological disorders .
Case Studies and Research Findings
Several studies have documented the applications of similar peptide compounds, providing insights into their efficacy and potential uses:
These findings underscore the compound's versatility and potential across various therapeutic domains.
Mechanism of Action
The mechanism of action of H-DL-Pyr-DL-His-DL-Trp-DL-Ser-OH depends on its specific application. In general, peptides exert their effects by binding to specific molecular targets, such as receptors or enzymes, and modulating their activity. The exact pathways involved can vary but often include signal transduction cascades and changes in gene expression.
Comparison with Similar Compounds
Structural and Functional Comparisons
The table below summarizes key structural and functional differences between H-DL-Pyr-DL-His-DL-Trp-DL-Ser-OH and related compounds:
*Calculated based on constituent amino acid molecular weights (Pyr: 129.1 g/mol, His: 155.2 g/mol, Trp: 204.2 g/mol, Ser: 105.1 g/mol) and peptide bond formation.
Key Differences
Sequence Complexity: The target compound’s tetrapeptide structure and inclusion of Pyr distinguish it from simpler dipeptides (e.g., H-Trp-Ser-OH) and tripeptides (e.g., H-Trp-Trp-Trp-OH).
Functional Groups: Unlike Formyl-DL-Trp-OH, which has a formylated N-terminus, the target retains a free amino group at the Pyr residue, altering solubility and reactivity .
Chirality : All residues in the target compound are DL-configured, whereas many analogs (e.g., H-Trp-Lys-OH) are typically synthesized in L-form for biological studies, impacting binding specificity .
Applications : While H-Trp-Trp-Trp-OH is used in fluorescence studies due to its triple indole rings, the target’s His residue may enable applications in metal-binding assays or pH-dependent studies .
Q & A
Q. What are the optimal synthetic routes for H-DL-Pyr-DL-His-DL-Trp-DL-Ser-OH, and what challenges arise due to its stereochemical complexity?
Basic Research Question
The synthesis of this tetrapeptide requires careful consideration of stereochemical control and protecting group strategies. Solid-phase peptide synthesis (SPPS) is commonly employed, but racemization risks at DL-configured residues (e.g., DL-Pyr, DL-His) necessitate low-temperature coupling and optimized activation reagents like HATU/HOAt . Challenges include:
- Racemization : Monitor via HPLC with chiral columns .
- Purification : Reverse-phase HPLC with gradients tailored to hydrophobic residues (e.g., Trp) .
- Yield optimization : Use orthogonal protecting groups (e.g., Fmoc for Ser, Boc for His) to minimize side reactions .
Q. How can researchers address discrepancies in bioactivity data across studies involving this compound?
Advanced Research Question
Data contradictions often stem from variability in experimental design or sample preparation. Methodological solutions include:
- Standardized protocols : Adopt uniform synthesis/purification criteria (e.g., ≥95% purity via HPLC) .
- Meta-analysis frameworks : Systematically compare studies using PRISMA guidelines to identify confounding variables (e.g., buffer composition, assay temperature) .
- Root-cause analysis : Apply Ishikawa diagrams to trace discrepancies to sources like oxidation of Trp residues or aggregation under specific pH conditions .
Q. What spectroscopic techniques are most effective for characterizing the tertiary structure of this compound?
Basic Research Question
- Circular Dichroism (CD) : Detects helical or β-sheet propensities in aqueous solutions; requires peptide concentrations ≥0.1 mg/mL .
- NMR Spectroscopy : 2D NOESY identifies inter-residue interactions; use D₂O or TFE for solubility .
- FT-IR : Monitors amide I/II bands to assess hydrogen bonding patterns .
Table 1: Comparison of Spectroscopic Techniques
Technique | Detection Limit | Key Application | Limitations |
---|---|---|---|
CD | 0.1 mg/mL | Secondary structure | Low resolution |
NMR | 1 mM | Tertiary interactions | Requires isotopic labeling |
FT-IR | 0.5 mg | Hydrogen bonding | Overlapping bands |
Q. What computational modeling approaches best predict the conformational dynamics of this compound in aqueous solutions?
Advanced Research Question
Molecular dynamics (MD) simulations using AMBER or CHARMM force fields are ideal. Key considerations:
- Solvation models : Explicit TIP3P water for accurate hydration effects .
- Sampling methods : Enhanced sampling (e.g., metadynamics) to overcome energy barriers in DL-residue folding .
- Validation : Compare simulated CD spectra with experimental data to refine force field parameters .
Q. How should researchers design controlled experiments to assess the stability of this compound under varying pH conditions?
Basic Research Question
- Experimental design :
- Controls : Include inert buffers (e.g., phosphate vs. Tris) to rule out buffer-specific effects .
Q. What strategies mitigate batch-to-batch variability in synthetic yields of this compound during scale-up experiments?
Advanced Research Question
- Process optimization :
- Statistical analysis : Apply factorial design (e.g., Taguchi method) to identify critical variables (e.g., resin swelling time, deprotection cycles) .
Table 2: Factors Influencing Synthetic Yield
Factor | Impact Level | Optimization Strategy |
---|---|---|
Resin type | High | Use low-swelling resins for DL-residues |
Activation time | Moderate | Limit to 2–5 minutes to reduce racemization |
Temperature | Critical | Maintain ≤4°C during coupling |
Q. How can researchers validate the biological relevance of this compound in cellular assays while minimizing off-target effects?
Advanced Research Question
- Assay design :
- Data interpretation : Apply stringent statistical thresholds (e.g., p<0.01, Bonferroni correction) to reduce false positives .
Q. What ethical and safety protocols are essential when handling this compound in laboratory settings?
Basic Research Question
Q. How do researchers integrate multi-omics data to elucidate the functional pathways modulated by this compound?
Advanced Research Question
- Methodology :
- Data integration : Use pathway enrichment tools (e.g., DAVID, STRING) to map cross-omics interactions .
Q. What criteria should guide the selection of animal models for in vivo studies of this compound?
Advanced Research Question
Properties
IUPAC Name |
3-hydroxy-2-[[2-[[3-(1H-imidazol-5-yl)-2-[(5-oxopyrrolidine-2-carbonyl)amino]propanoyl]amino]-3-(1H-indol-3-yl)propanoyl]amino]propanoic acid | |
---|---|---|
Details | Computed by Lexichem TK 2.7.0 (PubChem release 2021.05.07) | |
Source | PubChem | |
URL | https://pubchem.ncbi.nlm.nih.gov | |
Description | Data deposited in or computed by PubChem | |
InChI |
InChI=1S/C25H29N7O7/c33-11-20(25(38)39)32-23(36)18(7-13-9-27-16-4-2-1-3-15(13)16)30-24(37)19(8-14-10-26-12-28-14)31-22(35)17-5-6-21(34)29-17/h1-4,9-10,12,17-20,27,33H,5-8,11H2,(H,26,28)(H,29,34)(H,30,37)(H,31,35)(H,32,36)(H,38,39) | |
Details | Computed by InChI 1.0.6 (PubChem release 2021.05.07) | |
Source | PubChem | |
URL | https://pubchem.ncbi.nlm.nih.gov | |
Description | Data deposited in or computed by PubChem | |
InChI Key |
PLEVSTFISDCVMX-UHFFFAOYSA-N | |
Details | Computed by InChI 1.0.6 (PubChem release 2021.05.07) | |
Source | PubChem | |
URL | https://pubchem.ncbi.nlm.nih.gov | |
Description | Data deposited in or computed by PubChem | |
Canonical SMILES |
C1CC(=O)NC1C(=O)NC(CC2=CN=CN2)C(=O)NC(CC3=CNC4=CC=CC=C43)C(=O)NC(CO)C(=O)O | |
Details | Computed by OEChem 2.3.0 (PubChem release 2021.05.07) | |
Source | PubChem | |
URL | https://pubchem.ncbi.nlm.nih.gov | |
Description | Data deposited in or computed by PubChem | |
Molecular Formula |
C25H29N7O7 | |
Details | Computed by PubChem 2.1 (PubChem release 2021.05.07) | |
Source | PubChem | |
URL | https://pubchem.ncbi.nlm.nih.gov | |
Description | Data deposited in or computed by PubChem | |
Molecular Weight |
539.5 g/mol | |
Details | Computed by PubChem 2.1 (PubChem release 2021.05.07) | |
Source | PubChem | |
URL | https://pubchem.ncbi.nlm.nih.gov | |
Description | Data deposited in or computed by PubChem | |
Disclaimer and Information on In-Vitro Research Products
Please be aware that all articles and product information presented on BenchChem are intended solely for informational purposes. The products available for purchase on BenchChem are specifically designed for in-vitro studies, which are conducted outside of living organisms. In-vitro studies, derived from the Latin term "in glass," involve experiments performed in controlled laboratory settings using cells or tissues. It is important to note that these products are not categorized as medicines or drugs, and they have not received approval from the FDA for the prevention, treatment, or cure of any medical condition, ailment, or disease. We must emphasize that any form of bodily introduction of these products into humans or animals is strictly prohibited by law. It is essential to adhere to these guidelines to ensure compliance with legal and ethical standards in research and experimentation.