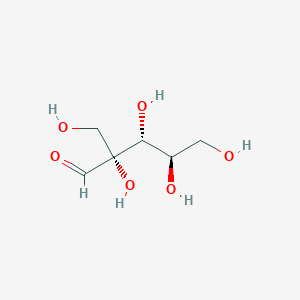
2-C-Hydroxymethyl-D-ribose
Overview
Description
Mechanism of Action
Target of Action
Hamamelose, also known as 2-C-(hydroxymethyl)-D-ribose, plays a significant role as a chiral building block in synthesizing a wide variety of enantiopure compounds . It is involved in the synthesis of L-hamamelose, a process that includes a stereoselective Grignard reaction, a stereoselective crossed aldol reaction, and a controlled oxidative cleavage of the double bond .
Mode of Action
Hamamelose interacts with its targets through a series of enzymatic reactions. In the metabolism of D-hamamelose, an enzyme known as RuBisCO-like protein (RLP) in Ochrobactrum anthropi ATCC 49188 (Oant_3067; HamA) functions as an oxygenase . This enzyme represents the first RLP catalyzing the O2-dependent oxidative C–C bond cleavage reaction .
Biochemical Pathways
The D-hamamelose pathway comprises five previously unknown enzymes: D-hamamelose dehydrogenase, D-hamamelono-lactonase, D-hamamelonate kinase, D-hamamelonate-2′,5-bisphosphate dehydrogenase (decarboxylating), and the RLP 3-keto-D-ribitol-1,5-bisphosphate (KRBP) oxygenase . This oxygenase converts KRBP to 3-D-phosphoglycerate and phosphoglycolate .
Pharmacokinetics
While specific ADME (Absorption, Distribution, Metabolism, and Excretion) properties of hamamelose are not readily available, it’s known that hamamelose kinase (EC 2.7.1.102) is an enzyme that catalyzes the chemical reaction of ATP and D-hamamelose to produce ADP and D-hamamelose 2’-phosphate . This suggests that hamamelose undergoes phosphorylation, a common process in the metabolism of many compounds.
Result of Action
Hamamelose has been shown to increase the susceptibility of Staphylococcus aureus biofilms towards antibiotics . It blocks the quorum sensing (QS) system through the TraP QS system, affecting cell wall synthesis and extracellular DNA release of S. aureus . After the enzymatic acylation of D-hamamelose, hamamelitannin, a bioactive natural substance, can be obtained .
Action Environment
The action of hamamelose can be influenced by various environmental factors. For instance, the enzymatic acylation of D-hamamelose with different vinyl gallates under the catalysis of Lipozyme TL IM in t-butyl alcohol (t-BuOH) or t-butyl methyl ether (t-BuMeO) provided different products . This suggests that the solvent used can influence the reaction outcome, indicating that the environment plays a crucial role in the action of hamamelose.
Biochemical Analysis
Biochemical Properties
Hamamelose is involved in several biochemical reactions, particularly in the catabolism and biosynthesis of sugars. It interacts with various enzymes, including glucose-6-phosphate isomerase and glucose-6-phosphate dehydrogenase . These interactions are crucial for the conversion of hamamelose into other metabolites, such as fructose 1-phosphate and fructose 1,6-bisphosphate, which are intermediates in glycolysis . The nature of these interactions involves the rearrangement of the carbon skeleton and phosphorylation, which are essential for the metabolic pathways in which hamamelose participates.
Cellular Effects
Hamamelose influences various cellular processes, including cell signaling pathways, gene expression, and cellular metabolism. It has been observed to affect the metabolic flux within cells, leading to changes in the levels of metabolites such as lactate, succinate, formate, acetate, and ethanol under anaerobic conditions . These effects are indicative of hamamelose’s role in cellular energy production and its impact on overall cell function.
Molecular Mechanism
The molecular mechanism of hamamelose involves its interaction with specific enzymes and biomolecules. For instance, hamamelose is converted into hamamelose 2-phosphate, which then undergoes further transformations to produce fructose 1-phosphate and fructose 1,6-bisphosphate . These reactions are facilitated by enzymes that recognize and bind to hamamelose, leading to its phosphorylation and subsequent breakdown. This process is crucial for the proper functioning of metabolic pathways and the regulation of gene expression related to these pathways.
Temporal Effects in Laboratory Settings
In laboratory settings, the effects of hamamelose have been studied over time to understand its stability, degradation, and long-term impact on cellular function. Studies have shown that hamamelose is relatively stable under various conditions, but its degradation can lead to the production of different metabolites depending on the environmental conditions . Long-term studies have indicated that hamamelose can influence cellular metabolism and energy production over extended periods, highlighting its potential for sustained biochemical activity.
Dosage Effects in Animal Models
The effects of hamamelose vary with different dosages in animal models. At lower doses, hamamelose has been observed to have beneficial effects on metabolic processes, while higher doses can lead to toxic or adverse effects . These threshold effects are important for determining the safe and effective use of hamamelose in various applications. Studies have shown that hamamelose can influence metabolic pathways and energy production in a dose-dependent manner, with higher doses potentially leading to metabolic imbalances.
Metabolic Pathways
Hamamelose is involved in several metabolic pathways, including glycolysis and the pentose phosphate pathway. It interacts with enzymes such as glucose-6-phosphate isomerase and glucose-6-phosphate dehydrogenase, which are crucial for its conversion into other metabolites . These interactions affect the metabolic flux within cells and can lead to changes in the levels of various metabolites. The involvement of hamamelose in these pathways highlights its importance in cellular metabolism and energy production.
Transport and Distribution
Within cells and tissues, hamamelose is transported and distributed through specific transporters and binding proteins. These interactions are essential for its localization and accumulation in different cellular compartments The transport and distribution of hamamelose can influence its activity and function, as well as its impact on cellular processes
Subcellular Localization
Hamamelose is localized in specific subcellular compartments, where it exerts its biochemical effects. It is directed to these compartments through targeting signals and post-translational modifications . The subcellular localization of hamamelose is important for its activity and function, as it allows for the precise regulation of metabolic pathways and cellular processes. This localization also influences the interactions of hamamelose with other biomolecules and its overall impact on cellular function.
Preparation Methods
Synthetic Routes and Reaction Conditions: The synthesis of 2-C-Hydroxymethyl-D-ribose typically involves the modification of D-ribose. One common method includes the attachment of an acetaldehyde group to the 2’-hydroxyl group on position C2 of ribose . This process is consistent with the observed 13C NMR spectrum, which shows peaks corresponding to the ribofuranoside moiety .
Industrial Production Methods: Industrial production of this compound is often carried out in cGMP (current Good Manufacturing Practice) synthesis workshops. These facilities ensure high-quality production standards, including cleanroom environments and rigorous quality assurance protocols.
Chemical Reactions Analysis
Types of Reactions: 2-C-Hydroxymethyl-D-ribose undergoes various chemical reactions, including oxidation, reduction, and substitution. The hydroxyl groups present in the compound make it susceptible to oxidation reactions, leading to the formation of corresponding aldehydes or carboxylic acids .
Common Reagents and Conditions: Common reagents used in these reactions include oxidizing agents like potassium permanganate and reducing agents such as sodium borohydride. The reaction conditions typically involve controlled temperatures and pH levels to ensure the desired product formation .
Major Products: The major products formed from these reactions include oxidized derivatives like 2-C-formyl-D-ribose and reduced forms such as 2-C-hydroxymethyl-D-ribitol .
Scientific Research Applications
2-C-Hydroxymethyl-D-ribose has a wide range of applications in scientific research:
Comparison with Similar Compounds
D-hamamelose:
Uniqueness: this compound is unique due to its hydroxymethyl group, which imparts distinct chemical properties and reactivity compared to other similar compounds. This uniqueness makes it highly valuable in pharmaceutical research and drug development.
Properties
IUPAC Name |
(2R,3R,4R)-2,3,4,5-tetrahydroxy-2-(hydroxymethyl)pentanal | |
---|---|---|
Source | PubChem | |
URL | https://pubchem.ncbi.nlm.nih.gov | |
Description | Data deposited in or computed by PubChem | |
InChI |
InChI=1S/C6H12O6/c7-1-4(10)5(11)6(12,2-8)3-9/h2,4-5,7,9-12H,1,3H2/t4-,5-,6-/m1/s1 | |
Source | PubChem | |
URL | https://pubchem.ncbi.nlm.nih.gov | |
Description | Data deposited in or computed by PubChem | |
InChI Key |
ZGVNGXVNRCEBDS-HSUXUTPPSA-N | |
Source | PubChem | |
URL | https://pubchem.ncbi.nlm.nih.gov | |
Description | Data deposited in or computed by PubChem | |
Canonical SMILES |
C(C(C(C(CO)(C=O)O)O)O)O | |
Source | PubChem | |
URL | https://pubchem.ncbi.nlm.nih.gov | |
Description | Data deposited in or computed by PubChem | |
Isomeric SMILES |
C([C@H]([C@H]([C@](CO)(C=O)O)O)O)O | |
Source | PubChem | |
URL | https://pubchem.ncbi.nlm.nih.gov | |
Description | Data deposited in or computed by PubChem | |
Molecular Formula |
C6H12O6 | |
Source | PubChem | |
URL | https://pubchem.ncbi.nlm.nih.gov | |
Description | Data deposited in or computed by PubChem | |
Molecular Weight |
180.16 g/mol | |
Source | PubChem | |
URL | https://pubchem.ncbi.nlm.nih.gov | |
Description | Data deposited in or computed by PubChem | |
CAS No. |
4573-78-8 | |
Record name | Hamamelose | |
Source | CAS Common Chemistry | |
URL | https://commonchemistry.cas.org/detail?cas_rn=4573-78-8 | |
Description | CAS Common Chemistry is an open community resource for accessing chemical information. Nearly 500,000 chemical substances from CAS REGISTRY cover areas of community interest, including common and frequently regulated chemicals, and those relevant to high school and undergraduate chemistry classes. This chemical information, curated by our expert scientists, is provided in alignment with our mission as a division of the American Chemical Society. | |
Explanation | The data from CAS Common Chemistry is provided under a CC-BY-NC 4.0 license, unless otherwise stated. | |
Record name | Hamamelose | |
Source | ChemIDplus | |
URL | https://pubchem.ncbi.nlm.nih.gov/substance/?source=chemidplus&sourceid=0004573788 | |
Description | ChemIDplus is a free, web search system that provides access to the structure and nomenclature authority files used for the identification of chemical substances cited in National Library of Medicine (NLM) databases, including the TOXNET system. | |
Record name | HAMAMELOSE | |
Source | FDA Global Substance Registration System (GSRS) | |
URL | https://gsrs.ncats.nih.gov/ginas/app/beta/substances/3107IMR9FU | |
Description | The FDA Global Substance Registration System (GSRS) enables the efficient and accurate exchange of information on what substances are in regulated products. Instead of relying on names, which vary across regulatory domains, countries, and regions, the GSRS knowledge base makes it possible for substances to be defined by standardized, scientific descriptions. | |
Explanation | Unless otherwise noted, the contents of the FDA website (www.fda.gov), both text and graphics, are not copyrighted. They are in the public domain and may be republished, reprinted and otherwise used freely by anyone without the need to obtain permission from FDA. Credit to the U.S. Food and Drug Administration as the source is appreciated but not required. | |
Retrosynthesis Analysis
AI-Powered Synthesis Planning: Our tool employs the Template_relevance Pistachio, Template_relevance Bkms_metabolic, Template_relevance Pistachio_ringbreaker, Template_relevance Reaxys, Template_relevance Reaxys_biocatalysis model, leveraging a vast database of chemical reactions to predict feasible synthetic routes.
One-Step Synthesis Focus: Specifically designed for one-step synthesis, it provides concise and direct routes for your target compounds, streamlining the synthesis process.
Accurate Predictions: Utilizing the extensive PISTACHIO, BKMS_METABOLIC, PISTACHIO_RINGBREAKER, REAXYS, REAXYS_BIOCATALYSIS database, our tool offers high-accuracy predictions, reflecting the latest in chemical research and data.
Strategy Settings
Precursor scoring | Relevance Heuristic |
---|---|
Min. plausibility | 0.01 |
Model | Template_relevance |
Template Set | Pistachio/Bkms_metabolic/Pistachio_ringbreaker/Reaxys/Reaxys_biocatalysis |
Top-N result to add to graph | 6 |
Feasible Synthetic Routes
Q1: What is the natural source of hamamelose?
A1: Hamamelose is found in almost all higher plants. It was first isolated from the bark of the witch hazel tree (Hamamelis virginiana L.). [, ]
Q2: How is hamamelose synthesized in plants?
A2: While the complete biosynthetic pathway is not fully elucidated, research suggests hamamelose is derived from chloroplastic fructose 1,6-bisphosphate. []
Q3: What is the metabolic fate of hamamelose in plants?
A3: Hamamelose can be converted to 2-carboxy-D-arabinitol (CA) and subsequently to 2-carboxy-D-arabinitol 1-phosphate (CA1P), a potent inhibitor of ribulose-1,5-bisphosphate carboxylase/oxygenase (RuBisCO), a key enzyme in photosynthesis. [, ]
Q4: Can microorganisms degrade hamamelose?
A4: Yes, certain microorganisms, like Kluyvera citrophila 627, can utilize hamamelose as a sole carbon source, catabolizing it through a pathway similar to glycolysis. [] Pseudomonas strain “H1” can oxidize hamamelose to hamamelonic acid. []
Q5: What is the molecular formula and weight of hamamelose?
A5: The molecular formula of hamamelose is C6H12O6, and its molecular weight is 180.156 g/mol.
Q6: What is the structure of hamamelose in solution?
A6: Hamamelose exists as an equilibrium mixture of two furanoses (five-membered ring) and two pyranoses (six-membered ring) in solution. []
Q7: What is the preferred conformation of hamamelose pyranoses?
A7: 1H-NMR data suggest that both hamamelose pyranoses favor the 1C4 conformation. []
Q8: How can hamamelose be chemically synthesized?
A8: Several synthetic routes have been developed, including:
- Isomerization of D-fructose in the presence of a molybdic acid catalyst. []
- From 2,2′-O-methylene-bis-D-glycerose through a series of aldol condensation, mercaptolysis, and demercaptalation reactions. []
- Reaction of 2,3-O-isopropylidene-D-ribose with formaldehyde, leading to the formation of 2,3-O-isopropylidene-D-hamamelose. []
Q9: Can hamamelose be chemically modified?
A9: Yes, hamamelose can be chemically modified. For example, acylation reactions can introduce galloyl groups, leading to the synthesis of compounds like hamamelitannin (2′,5-di-O-galloyl-d-hamamelose). []
Q10: What is hamamelitannin, and what are its potential applications?
A10: Hamamelitannin is a galloylated derivative of hamamelose found in witch hazel bark. It exhibits various biological activities, including antioxidant, anti-inflammatory, and antimicrobial properties. [, , , ] It has shown potential as a quorum-sensing inhibitor, particularly against Staphylococcus aureus, making it a promising candidate for developing new treatments for drug-resistant bacterial infections. [, ]
Q11: Can structural modifications of hamamelose derivatives affect their activity?
A11: Yes, the number and position of phenolic groups, such as galloyl groups, significantly influence the biological activity of hamamelose derivatives. For instance, tetragalloyl glucose exhibits higher apoptosis-inducing activity than digalloyl hamamelose and monogalloyl α-glucoside. []
Q12: What is the role of hamamelose in photosynthesis regulation?
A12: Hamamelose is a precursor to CA1P, which acts as a nocturnal inhibitor of RuBisCO. This suggests a role for hamamelose in the diurnal regulation of photosynthesis. []
Disclaimer and Information on In-Vitro Research Products
Please be aware that all articles and product information presented on BenchChem are intended solely for informational purposes. The products available for purchase on BenchChem are specifically designed for in-vitro studies, which are conducted outside of living organisms. In-vitro studies, derived from the Latin term "in glass," involve experiments performed in controlled laboratory settings using cells or tissues. It is important to note that these products are not categorized as medicines or drugs, and they have not received approval from the FDA for the prevention, treatment, or cure of any medical condition, ailment, or disease. We must emphasize that any form of bodily introduction of these products into humans or animals is strictly prohibited by law. It is essential to adhere to these guidelines to ensure compliance with legal and ethical standards in research and experimentation.