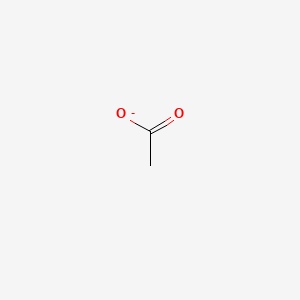
Acetate
Overview
Description
Acetate is a chemical compound with the formula C₂H₃O₂⁻. It is the conjugate base or ion of acetic acid, typically found in aqueous solutions. This compound is a versatile compound that forms salts and esters with various cations. It is widely used in both industrial and biological processes. In nature, this compound is a common building block for biosynthesis.
Preparation Methods
Synthetic Routes and Reaction Conditions
Acetate can be synthesized through several methods. One common method involves the reaction of acetic acid with a base, such as sodium hydroxide, to form sodium this compound and water:
CH3COOH+NaOH→CH3COONa+H2O
Another method involves the esterification of acetic acid with ethanol in the presence of a catalyst like sulfuric acid to produce ethyl this compound:
CH3COOH+C2H5OH→CH3COOC2H5+H2O
Industrial Production Methods
Industrially, this compound is produced on a large scale through the carbonylation of methanol, which involves the reaction of methanol with carbon monoxide in the presence of a catalyst to form acetic acid, which is then converted to this compound salts or esters .
Chemical Reactions Analysis
Types of Reactions
Acetate undergoes various chemical reactions, including:
Oxidation: this compound can be oxidized to carbon dioxide and water.
Reduction: this compound can be reduced to ethanol.
Substitution: this compound can undergo nucleophilic substitution reactions to form different esters.
Common Reagents and Conditions
Oxidation: Potassium permanganate (KMnO₄) or hydrogen peroxide (H₂O₂) can be used as oxidizing agents.
Reduction: Lithium aluminum hydride (LiAlH₄) is commonly used as a reducing agent.
Substitution: Acid chlorides or anhydrides can be used in the presence of a base to form esters.
Major Products
Oxidation: Carbon dioxide (CO₂) and water (H₂O).
Reduction: Ethanol (C₂H₅OH).
Substitution: Various esters, such as ethyl this compound (CH₃COOC₂H₅).
Scientific Research Applications
Acetate has numerous applications in scientific research:
Chemistry: Used as a solvent and reagent in organic synthesis.
Biology: Plays a role in metabolic pathways, such as the citric acid cycle.
Medicine: Used in the formulation of pharmaceuticals and as a buffer in biological systems.
Industry: Used in the production of polymers, food preservatives, and as a solvent in coatings and inks .
Mechanism of Action
Acetate exerts its effects through various mechanisms:
Metabolic Pathways: this compound is converted to acetyl-CoA, which enters the citric acid cycle to produce energy.
Buffering Action: this compound acts as a buffer, maintaining pH levels in biological systems.
Enzyme Activation: This compound can activate enzymes involved in metabolic processes .
Comparison with Similar Compounds
Similar Compounds
Formate (HCOO⁻): Similar to acetate but with one less carbon atom.
Propionate (C₃H₅O₂⁻): Similar to this compound but with one additional carbon atom.
Butyrate (C₄H₇O₂⁻): Similar to this compound but with two additional carbon atoms.
Uniqueness of this compound
Versatility: this compound can form a wide range of salts and esters.
Biological Importance: this compound is a key intermediate in metabolic pathways.
Industrial Relevance: This compound is used in various industrial applications, from solvents to food preservatives .
Properties
CAS No. |
71-50-1 |
---|---|
Molecular Formula |
C2H3O2- |
Molecular Weight |
59.04 g/mol |
IUPAC Name |
acetate |
InChI |
InChI=1S/C2H4O2/c1-2(3)4/h1H3,(H,3,4)/p-1 |
InChI Key |
QTBSBXVTEAMEQO-UHFFFAOYSA-M |
SMILES |
CC(=O)[O-] |
Canonical SMILES |
CC(=O)[O-] |
Key on ui other cas no. |
71-50-1 |
Origin of Product |
United States |
Synthesis routes and methods I
Procedure details
Synthesis routes and methods II
Procedure details
Synthesis routes and methods III
Procedure details
Synthesis routes and methods IV
Procedure details
Retrosynthesis Analysis
AI-Powered Synthesis Planning: Our tool employs the Template_relevance Pistachio, Template_relevance Bkms_metabolic, Template_relevance Pistachio_ringbreaker, Template_relevance Reaxys, Template_relevance Reaxys_biocatalysis model, leveraging a vast database of chemical reactions to predict feasible synthetic routes.
One-Step Synthesis Focus: Specifically designed for one-step synthesis, it provides concise and direct routes for your target compounds, streamlining the synthesis process.
Accurate Predictions: Utilizing the extensive PISTACHIO, BKMS_METABOLIC, PISTACHIO_RINGBREAKER, REAXYS, REAXYS_BIOCATALYSIS database, our tool offers high-accuracy predictions, reflecting the latest in chemical research and data.
Strategy Settings
Precursor scoring | Relevance Heuristic |
---|---|
Min. plausibility | 0.01 |
Model | Template_relevance |
Template Set | Pistachio/Bkms_metabolic/Pistachio_ringbreaker/Reaxys/Reaxys_biocatalysis |
Top-N result to add to graph | 6 |
Feasible Synthetic Routes
Disclaimer and Information on In-Vitro Research Products
Please be aware that all articles and product information presented on BenchChem are intended solely for informational purposes. The products available for purchase on BenchChem are specifically designed for in-vitro studies, which are conducted outside of living organisms. In-vitro studies, derived from the Latin term "in glass," involve experiments performed in controlled laboratory settings using cells or tissues. It is important to note that these products are not categorized as medicines or drugs, and they have not received approval from the FDA for the prevention, treatment, or cure of any medical condition, ailment, or disease. We must emphasize that any form of bodily introduction of these products into humans or animals is strictly prohibited by law. It is essential to adhere to these guidelines to ensure compliance with legal and ethical standards in research and experimentation.