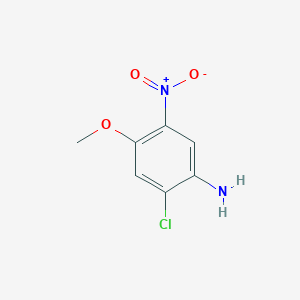
2-Chloro-4-methoxy-5-nitroaniline
- Click on QUICK INQUIRY to receive a quote from our team of experts.
- With the quality product at a COMPETITIVE price, you can focus more on your research.
Overview
Description
2-Chloro-4-methoxy-5-nitroaniline is an organic compound with the molecular formula C7H7ClN2O3 It is a derivative of aniline, characterized by the presence of chloro, methoxy, and nitro substituents on the benzene ring
Preparation Methods
Synthetic Routes and Reaction Conditions
-
Nitration of 2-Chloro-4-methoxyaniline: : The synthesis of 2-Chloro-4-methoxy-5-nitroaniline typically begins with the nitration of 2-Chloro-4-methoxyaniline. This reaction is carried out using a mixture of concentrated nitric acid and sulfuric acid at low temperatures to control the reaction rate and prevent over-nitration. The reaction proceeds as follows:
2-Chloro-4-methoxyaniline+HNO3→this compound+H2O
-
Chlorination of 4-Methoxy-5-nitroaniline: : Another method involves the chlorination of 4-Methoxy-5-nitroaniline using chlorine gas or a chlorinating agent like thionyl chloride. This reaction is typically conducted in the presence of a catalyst such as iron(III) chloride to facilitate the substitution of the hydrogen atom with a chlorine atom.
Industrial Production Methods
In industrial settings, the production of this compound is often scaled up using continuous flow reactors to ensure consistent quality and yield. The use of automated systems allows for precise control of reaction conditions, such as temperature, pressure, and reagent concentrations, which is crucial for large-scale synthesis.
Chemical Reactions Analysis
Types of Reactions
-
Reduction: : 2-Chloro-4-methoxy-5-nitroaniline can undergo reduction reactions to form 2-Chloro-4-methoxy-5-aminoaniline. This reaction is typically carried out using reducing agents such as hydrogen gas in the presence of a palladium catalyst or using chemical reducing agents like tin(II) chloride in hydrochloric acid.
-
Substitution: : The compound can participate in nucleophilic substitution reactions, where the chloro group can be replaced by other nucleophiles such as amines, thiols, or alkoxides. These reactions are often facilitated by the presence of a base such as sodium hydroxide or potassium carbonate.
Common Reagents and Conditions
Reducing Agents: Hydrogen gas with palladium catalyst, tin(II) chloride in hydrochloric acid.
Nucleophiles: Amines, thiols, alkoxides.
Catalysts: Iron(III) chloride for chlorination, palladium for reduction.
Major Products
Reduction: 2-Chloro-4-methoxy-5-aminoaniline.
Substitution: Various substituted anilines depending on the nucleophile used.
Scientific Research Applications
Chemistry
In organic synthesis, 2-Chloro-4-methoxy-5-nitroaniline is used as an intermediate for the preparation of more complex molecules. Its functional groups allow for further chemical modifications, making it a valuable building block in the synthesis of dyes, pigments, and pharmaceuticals.
Biology and Medicine
In medicinal chemistry, derivatives of this compound have been investigated for their potential biological activities. These compounds may exhibit antimicrobial, antifungal, or anticancer properties, making them candidates for drug development.
Industry
In the materials science industry, this compound is used in the production of specialty polymers and resins. Its unique chemical structure imparts desirable properties such as thermal stability and resistance to degradation, which are important for high-performance materials.
Mechanism of Action
The biological activity of 2-Chloro-4-methoxy-5-nitroaniline and its derivatives is often attributed to their ability to interact with specific molecular targets, such as enzymes or receptors. The nitro group can undergo bioreduction to form reactive intermediates that can interact with cellular components, leading to various biological effects. The chloro and methoxy groups can influence the compound’s lipophilicity and ability to cross cell membranes, affecting its distribution and activity within the body.
Comparison with Similar Compounds
Similar Compounds
2-Chloro-4-methoxyaniline: Lacks the nitro group, making it less reactive in certain chemical reactions.
4-Methoxy-5-nitroaniline: Lacks the chloro group, which can affect its chemical reactivity and biological activity.
2-Chloro-5-nitroaniline: Lacks the methoxy group, which can influence its solubility and interaction with biological targets.
Uniqueness
2-Chloro-4-methoxy-5-nitroaniline is unique due to the presence of all three functional groups (chloro, methoxy, and nitro) on the benzene ring. This combination of substituents provides a balance of reactivity and stability, making it a versatile compound for various applications in research and industry.
Biological Activity
2-Chloro-4-methoxy-5-nitroaniline (C7H7ClN2O3) is a nitroaniline compound that has garnered attention for its biological activity, particularly in the fields of environmental science and toxicology. This article provides a comprehensive overview of the biological activities associated with this compound, including its mechanisms of action, metabolic pathways, and potential toxicological effects.
Chemical Structure and Properties
This compound is characterized by the presence of a chloro group, methoxy group, and nitro group attached to an aniline structure. This unique arrangement contributes to its reactivity and biological interactions.
The biological activity of this compound can be attributed to several mechanisms:
- Enzyme Interaction : The compound interacts with various enzymes, notably flavin-dependent monooxygenases, which facilitate the removal of nitro groups, leading to the formation of metabolites such as 4-amino-3-chlorophenol (4-A-3-CP). These transformations are crucial for understanding its degradation and potential toxicity .
- Cellular Effects : At the cellular level, exposure to this compound can induce oxidative stress, affecting cellular signaling pathways and gene expression related to stress responses. High concentrations have been linked to cellular damage and alterations in metabolic processes .
- Mutagenicity : Studies indicate that compounds with nitro groups in specific positions exhibit mutagenic activity. This compound has demonstrated mutagenic potential in bacterial assays, particularly when metabolic activation is involved .
Case Studies
- Subacute Toxicity in Rodents : Research involving B6C3F1/N mice exposed to varying concentrations of related nitroanilines showed significant adverse effects on liver and kidney weights at high doses (up to 10,000 ppm). Histopathological evaluations revealed changes consistent with toxicity, including increased hemosiderin pigmentation in the liver and spleen .
- Reproductive Toxicity : A reproductive toxicity study indicated that exposure during gestation affected both maternal health and offspring viability. Notably, maternal weight loss and altered organ weights were observed at high exposure levels .
Metabolic Pathways
The metabolism of this compound involves several key enzymes:
- Flavin-dependent Monooxygenases : These enzymes initiate the degradation process by converting the compound into less toxic metabolites.
- Aniline Dioxygenases : Involved in subsequent steps of metabolism, contributing to the breakdown of aromatic compounds into simpler forms that can be excreted or further metabolized .
Environmental Impact
The degradation pathways of this compound have implications for environmental remediation efforts. Strain MB-P1, a bacterium capable of utilizing this compound as a carbon source, has been studied for its potential to bioremediate contaminated environments by breaking down nitroaromatic compounds into less harmful substances .
Data Summary
Properties
Molecular Formula |
C7H7ClN2O3 |
---|---|
Molecular Weight |
202.59 g/mol |
IUPAC Name |
2-chloro-4-methoxy-5-nitroaniline |
InChI |
InChI=1S/C7H7ClN2O3/c1-13-7-2-4(8)5(9)3-6(7)10(11)12/h2-3H,9H2,1H3 |
InChI Key |
BCQRKWDKIJWZRN-UHFFFAOYSA-N |
Canonical SMILES |
COC1=C(C=C(C(=C1)Cl)N)[N+](=O)[O-] |
Origin of Product |
United States |
Disclaimer and Information on In-Vitro Research Products
Please be aware that all articles and product information presented on BenchChem are intended solely for informational purposes. The products available for purchase on BenchChem are specifically designed for in-vitro studies, which are conducted outside of living organisms. In-vitro studies, derived from the Latin term "in glass," involve experiments performed in controlled laboratory settings using cells or tissues. It is important to note that these products are not categorized as medicines or drugs, and they have not received approval from the FDA for the prevention, treatment, or cure of any medical condition, ailment, or disease. We must emphasize that any form of bodily introduction of these products into humans or animals is strictly prohibited by law. It is essential to adhere to these guidelines to ensure compliance with legal and ethical standards in research and experimentation.