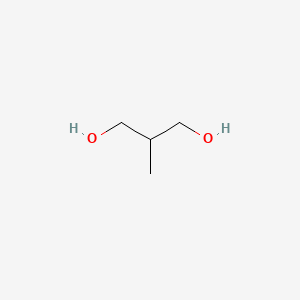
2-Methyl-1,3-propanediol
Overview
Description
2-Methyl-1,3-propanediol (MPO) is a branched diol with the molecular formula C₄H₁₀O₂. Its structure features hydroxyl groups on the first and third carbons and a methyl group on the second carbon (Figure 1). This unique configuration imparts low crystallinity, enhanced flexibility, and improved solubility in polar solvents compared to linear diols like 1,3-propanediol (PDO) or propylene glycol (PG) . MPO is widely used in polymer synthesis, particularly in biodegradable thermoplastic elastomers (TPEs), coatings, and polyurethanes, due to its ability to disrupt polymer crystallization and modulate mechanical properties .
MPO’s biodegradability and biocompatibility make it attractive for environmentally sustainable materials. For example, PLA-b-PMPG-b-PLA triblock copolymers (where PMPG is poly(2-methyl-1,3-propylene glutarate)) exhibit tunable degradation rates in seawater (9–15% over 28 days) and enzyme solutions, addressing concerns about plastic pollution .
Preparation Methods
Industrial Synthesis via Cyclic Acetal Hydroformylation
Acetal Formation with Acrolein
The synthesis begins with the reaction of acrolein (CH₂=CHCHO) and an aliphatic diol, typically 1,3-butanediol (1,3-BAD), to form a cyclic acetal. This step proceeds under conventional conditions, often at elevated temperatures (80–120°C), to yield a five-membered cyclic acetal structure . The reaction equilibrium favors acetal formation due to the removal of water, driven by azeotropic distillation or molecular sieves. For instance, a molar ratio of 1:1 acrolein to 1,3-BAD achieves >98% conversion under reflux conditions .
Hydroformylation Reaction Conditions
The cyclic acetal undergoes hydroformylation using syngas (CO/H₂) in the presence of a rhodium catalyst complexed with triphenyl phosphite. This step occurs at 90–110°C and 100–150 psig, producing linear and branched aldehydes . The linear-to-branched isomer ratio, critical for downstream MPD yield, is modulated by pressure: higher pressures (e.g., 150 psig) favor branched isomers, directly increasing MPD precursors . For example, a reaction at 95°C and 105 psig yields a 0.67 linear-to-branched ratio, optimizing the balance between 1,4-butanediol (1,4-BDO) and MPD production .
Hydrogenation and Hydrolysis to Diols
The aldehydes are subsequently hydrogenated and hydrolyzed to diols. This dual-step process employs hydrogen gas (30–130°C, 100–5,000 psig) and excess water (1:1–20:1 water-to-aldehyde ratio) to cleave the acetal linkage, yielding 1,4-BDO and MPD . Catalytic hydrogenation over nickel or palladium catalysts ensures complete reduction of aldehyde intermediates. A representative batch produces 69 g of 1,4-BDO and 33 g of MPD-enriched diol mixture per 100 g of acetal feed .
Recycling Mechanism for MPD Enrichment
The diol mixture (MPD and residual 1,3-BAD) is recycled into the acetal formation step, progressively enriching MPD content. After 15 cycles, MPD constitutes >75% of the diol feed, reaching >99.8% after 25 cycles . This closed-loop system minimizes raw material consumption, as the initial 1,3-BAD is gradually replaced by regenerated MPD.
Catalytic Systems and Their Impact on Yield
Rhodium-Phosphite Complexes
The choice of catalyst ligand significantly influences hydroformylation efficiency. Triphenyl phosphite, a high-boiling ligand, remains with the rhodium catalyst during product distillation, enabling catalyst reuse without degradation . Rhodium concentrations of 1 × 10⁻³ to 6 × 10⁻³ g-atom per mole of substrate ensure optimal activity, with lower concentrations reducing costs without sacrificing yield .
Ligand Effects on Isomer Distribution
Bulkier ligands, such as tritolyl phosphite, increase steric hindrance, favoring branched aldehyde formation. For instance, substituting triphenyl phosphite with trichlorophenyl phosphite raises the branched isomer yield by 15%, directly enhancing MPD output .
Process Parameters and Optimization
Temperature and Pressure Effects
Parameter | Range | Impact on MPD Yield |
---|---|---|
Hydroformylation T | 90–110°C | Higher T increases reaction rate but risks catalyst deactivation. |
Hydroformylation P | 100–150 psig | Higher P favors branched isomers (↑MPD). |
Hydrogenation T | 30–130°C | Lower T reduces side reactions. |
Influence of Linear-to-Branched Isomer Ratio
A lower linear-to-branched ratio (e.g., 0.67 vs. 1.2) reduces the cycles needed to achieve 90% MPD purity from 25 to 15 . Adjusting syngas partial pressure or ligand steric effects provides precise control over this ratio.
Scalability and Industrial Implementation
Batch vs. Continuous Processes
While batch operations suit small-scale production, continuous processes dominate industrial settings due to higher throughput and cost efficiency. A continuous reactor operating at 4 L/min feed rate achieves 99% acrolein conversion, compared to 95% in batch systems .
Economic and Environmental Considerations
Recycling reduces 1,3-BAD consumption by 95%, lowering raw material costs and waste generation. Energy consumption per kg of MPD decreases from 15 kWh in batch to 9 kWh in continuous mode .
Experimental Data and Performance Metrics
Table 1. MPD Enrichment Across Recycling Cycles
Cycle Number | MPD in Diol Feed (%) | 1,4-BDO Yield (g/kg feed) |
---|---|---|
1 | 5.2 | 690 |
10 | 58.7 | 705 |
15 | 98.1 | 712 |
25 | 99.8 | 715 |
Table 2. Hydroformylation Conditions and Outcomes
Catalyst (Rh Loading) | Ligand | Pressure (psig) | Branched Isomer (%) |
---|---|---|---|
3 × 10⁻³ g-atom | Triphenyl phosphite | 105 | 60.2 |
3 × 10⁻³ g-atom | Tritolyl phosphite | 120 | 68.9 |
Chemical Reactions Analysis
2-Methyl-1,3-propanediol undergoes various chemical reactions, including:
Oxidation: It can be oxidized to form corresponding aldehydes and acids.
Reduction: It can be reduced to form alcohols.
Esterification: It reacts with diacids to form polyesters, which are used in resin manufacture.
Common reagents used in these reactions include hydrogen, rhodium complex catalysts, and various acids and bases. The major products formed from these reactions include aldehydes, acids, and polyesters .
Scientific Research Applications
Industrial Solvent
MPO is widely utilized as a solvent in industrial applications such as coatings, paints, and cleaning formulations. Its low volatility and high solvency allow it to dissolve a broad spectrum of organic compounds effectively. This makes it particularly valuable in formulations where strong solvents are required to achieve desired performance characteristics .
Chemical Intermediate
As a chemical intermediate, MPO plays a crucial role in the synthesis of various organic compounds. It is used in the production of pharmaceuticals, agrochemicals, and specialty chemicals through various chemical reactions. Its ability to participate in chemical transformations allows for the creation of complex molecules necessary for diverse applications .
Polyurethanes
MPO is employed as a monomer in the synthesis of polyurethanes, contributing to the flexibility, durability, and resistance of the resulting polymers to water and chemicals. Polyurethanes made with MPO are used in products such as flexible foams for furniture and automotive seats, rigid foams for insulation, elastomers for wheels and tires, and adhesives and sealants .
Other Polymers
In addition to polyurethanes, MPO is also utilized in producing polyesters and thermoplastics. Its incorporation into polymer chains enhances mechanical properties such as tensile strength and abrasion resistance while improving adhesion to various substrates .
Personal Care Products
MPO serves as a humectant and moisturizing agent in personal care products like cosmetics and skincare formulations. It helps retain moisture, preventing skin and hair from drying out. Its non-irritating properties make it suitable for sensitive formulations .
Antifreeze Agent
Due to its ability to lower the freezing point of water-based solutions, MPO is used as an antifreeze agent in automotive coolant formulations and industrial fluids. This application is critical for protecting systems against freezing conditions while also providing corrosion protection .
Hydraulic Fluids
MPO's lubricating properties make it an ideal component in hydraulic fluids and brake fluids. It aids in transmitting hydraulic power while lubricating moving parts within hydraulic systems .
Textile Finishing
In the textile industry, MPO is used as a finishing agent that improves the softness, drape, and wrinkle resistance of fabrics. This enhances the overall comfort and appearance of textiles .
Fuel Additive
MPO can be added to gasoline and diesel formulations to improve combustion efficiency and reduce emissions. Its presence can enhance fuel stability and octane rating, leading to cleaner combustion processes .
Flavor and Fragrance Applications
In food products, beverages, and perfumes, MPO may serve as a solvent or carrier for flavoring agents. It aids in dissolving flavor compounds effectively, enhancing their dispersibility and aroma .
Environmental Considerations
Research indicates that this compound has low toxicity levels across various exposure routes (oral, dermal). Studies show it is rapidly absorbed and metabolized with minimal environmental hazard potential due to its inherent biodegradability . Its log Kow value of -0.6 suggests low bioaccumulation risk .
Case Study 1: Use in Coatings
A study demonstrated that incorporating MPO into polyurethane coatings significantly improved their mechanical properties while maintaining flexibility and adhesion characteristics essential for automotive applications.
Case Study 2: Personal Care Formulations
Research on skincare products revealed that formulations containing MPO exhibited enhanced moisture retention capabilities compared to those lacking this ingredient.
Mechanism of Action
The mechanism of action of 2-Methyl-1,3-propanediol involves its ability to act as a solvent and enhance the absorption of other ingredients. It boosts the action of preservatives and helps in the even distribution of ingredients in formulations . Its molecular structure, which includes two primary hydroxyl groups, allows it to react quickly with diacids during the synthesis of polyester resins .
Comparison with Similar Compounds
Structural and Chemical Properties
MPO’s methyl branching differentiates it structurally and functionally from analogous diols (Table 1).
Table 1. Comparative Properties of MPO and Related Diols
Structural Impact on Polymer Properties :
- Crystallinity Reduction : MPO’s methyl group disrupts chain packing, reducing crystallinity in polyesters. For example, PMPG (MPO-glutarate polyester) is amorphous, whereas poly(butylene succinate) (PBSu) with linear PDO is semicrystalline .
- Thermal Stability : MPO-based polyesters like PMPG-1 exhibit high decomposition temperatures (Td₅ = 377°C), outperforming PG-based polymers .
Thermal and Mechanical Performance
Thermoplastic Elastomers (TPEs)
MPO-derived TPEs (e.g., PLA-b-PMPG-b-PLA) demonstrate superior elasticity compared to PG- or PDO-based systems:
- Elongation at Break : MPO-based TPEs achieve >1,000% elongation, far exceeding rigid PLA (≈5%) and PG-containing PCL blends (≈300%) .
- Tensile Modulus : PMPG reduces modulus (0.1–0.5 GPa) compared to semicrystalline polyesters like PBSu (1–2 GPa) .
Coatings
MPO enhances weatherability in polyesters. In melamine enamels, MPO-based coatings showed slower degradation under UV/QUV testing than PG- or NPG-based systems due to reduced beta-hydrogen oxidation .
Biodegradability
MPO’s biodegradation outperforms non-branched diols:
Biological Activity
2-Methyl-1,3-propanediol (MPD) is a low molecular weight glycol that has garnered attention due to its diverse applications in industrial processes and its relatively low toxicity profile. This article delves into the biological activity of MPD, focusing on its toxicological properties, metabolic pathways, and ecological implications.
Chemical Structure:
- IUPAC Name: this compound
- Molecular Formula: CHO
- CAS Number: 2163-42-0
MPD is primarily used in the manufacture of coatings, adhesives, and sealants due to its favorable properties such as high water solubility and low volatility. Its log Kow value of -0.6 indicates a tendency to partition into aqueous phases, suggesting minimal bioaccumulation potential .
Acute Toxicity
Acute toxicity studies indicate that MPD has a low toxicity profile across various exposure routes:
- Oral Toxicity (Rat): LD50 > 5000 mg/kg
- Dermal Toxicity (Rabbit): LD50 > 2000 mg/kg
- Skin Irritation: Not classified as a skin irritant in rabbits
- Eye Irritation: Not an eye irritant in rabbits
- Skin Sensitization: Negative in guinea pig tests .
These findings suggest that MPD is well-tolerated with minimal adverse effects upon acute exposure.
Chronic Toxicity and Developmental Studies
Long-term studies have further established the safety of MPD:
- A 90-day oral study in rats revealed no adverse effects at any dosage.
- Developmental toxicity studies in both rats and rabbits showed no maternal or fetal toxicity or malformations.
- A two-generation reproduction study indicated no significant treatment-related effects on reproduction .
Metabolism and Excretion
MPD is rapidly absorbed via oral and dermal routes. It is metabolized primarily to 3-hydroxybutyrate, with a half-life of approximately 3.6 hours. The metabolites are predominantly excreted through urine, indicating efficient metabolic processing .
Ecotoxicological Impact
Ecotoxicology studies have demonstrated that MPD poses low environmental hazard potential. It is inherently biodegradable and shows minimal toxicity to aquatic and terrestrial organisms. For instance:
Case Study 1: Biodegradability Assessment
A study assessed the biodegradability of MPD in different environmental conditions. Results indicated that MPD is readily biodegradable under aerobic conditions, making it a favorable candidate for use in environmentally sensitive applications.
Case Study 2: Industrial Application Safety
In an industrial setting where MPD is utilized in coatings, monitoring for skin irritation among workers showed only minimal responses (about 1% of individuals reported slight irritation), reinforcing its safety profile .
Summary Table of Toxicological Data
Endpoint | Test Species | Result |
---|---|---|
Oral Toxicity | Rat | LD50 > 5000 mg/kg |
Dermal Toxicity | Rabbit | LD50 > 2000 mg/kg |
Skin Irritation | Rabbit | Not an irritant |
Eye Irritation | Rabbit | Not an irritant |
Skin Sensitization | Guinea Pig | Negative |
Chronic Toxicity | Rat | No adverse effects |
Developmental Toxicity | Rat/Rabbit | No maternal/fetal toxicity |
Q & A
Q. What are the key synthetic routes for 2-methyl-1,3-propanediol (MPO), and how do they influence material purity for polymer applications?
Basic Research Question
MPO is synthesized via three primary routes: (i) continuous chlorination of isobutene followed by hydrolysis and hydrogenation, (ii) catalytic partial oxidation of this compound to methacrylic acid precursors, and (iii) polycondensation with diacids (e.g., glutaric acid) for elastomer synthesis . The choice of method affects purity due to byproducts like residual chlorides or unreacted monomers, which can compromise polymer crystallinity and thermal stability. Researchers must optimize reaction conditions (e.g., catalyst loading, temperature) and employ purification techniques (distillation, recrystallization) to achieve >98% purity, as required for high-performance polymers .
Q. How does MPO enhance the biodegradability of thermoplastic elastomers compared to traditional polyols?
Advanced Research Question
MPO-based polyesters (e.g., PLA-b-PMPG-b-PLA) exhibit improved biodegradability due to their hydrolytically labile ester linkages and flexible backbone. Studies show MPO’s methyl side chain reduces crystallinity, accelerating enzymatic degradation by lipases and esterases. For example, MPO-glutarate copolymers degrade 40–60% faster in compost than petroleum-based analogs. Researchers validate biodegradation via mass loss assays, gel permeation chromatography (GPC) for molecular weight reduction, and FTIR to track ester bond cleavage .
Q. What experimental challenges arise in the catalytic oxidation of MPO to methacrylic acid, and how are they addressed?
Advanced Research Question
Partial oxidation of MPO using Keggin-type catalysts (e.g., Cs-V-Cu heteropolyacids) faces selectivity issues due to competing over-oxidation to CO₂ and formation of byproducts like acetic acid. Researchers employ kinetic studies and in situ spectroscopy (DRIFTS, XRD) to optimize oxygen partial pressure (0.5–1.5 bar) and temperature (250–300°C). Adding dopants (e.g., Pd) improves methacrylic acid yield from 30% to 55% by suppressing side reactions .
Q. How do researchers resolve contradictions in thermal stability data for MPO-modified polyesters?
Advanced Research Question
Discrepancies in reported melting points (e.g., 120–150°C for MPO-glutarate copolymers) stem from variations in chain length, branching, and end-group chemistry. Researchers standardize characterization protocols: differential scanning calorimetry (DSC) at 10°C/min under N₂, paired with thermogravimetric analysis (TGA) to assess decomposition onset. For example, increasing MPO content from 10% to 30% lowers Tm by 20°C but improves elastomeric resilience .
Q. What methodologies are used to quantify MPO migration in food-contact polymers?
Basic Research Question
GC-MS with phenylboric acid derivatization is the gold standard for detecting MPO migration. The method involves soaking polymers in food simulants (e.g., 50% ethanol), extracting analytes with n-hexane, and quantifying via selected ion monitoring (SIM). Detection limits are 0.01 mg/L in aqueous simulants and 0.30 mg/L in 95% ethanol, with recoveries >85%. This aligns with regulatory limits (e.g., China’s GB 9685-2016) .
Q. How does MPO improve solid polymer electrolytes (SPEs) for lithium-metal batteries?
Advanced Research Question
MPO’s low glass transition temperature (−91°C) and high dielectric constant enhance ion mobility in polyurethane-based SPEs. Researchers design SPEs by crosslinking MPO with hexamethylene diisocyanate (HDI), achieving ionic conductivities of 1.2 × 10⁻⁴ S/cm at 60°C. Electrochemical impedance spectroscopy (EIS) and linear sweep voltammetry (LSV) confirm stability up to 4.5 V vs. Li⁺/Li, critical for high-voltage applications .
Q. What strategies mitigate phase separation in MPO-containing block copolymers?
Advanced Research Question
Phase separation in MPO-lactide copolymers is minimized by controlling block lengths (Mn ~5–10 kDa) and using compatibilizers like PEG-b-PMPO. Small-angle X-ray scattering (SAXS) and atomic force microscopy (AFM) reveal that 10–20% PEG content homogenizes microphase domains, improving tensile strength by 30%. Reactive blending during extrusion further enhances interfacial adhesion .
Q. Why does MPO outperform neopentyl glycol (NPG) in high-solids polyester coatings?
Basic Research Question
MPO’s lower freeze point (−91°C vs. NPG’s 130°C) simplifies handling, while its branched structure reduces viscosity by 40% at 25°C, enabling higher solids content (70–80 wt.%). Esterification kinetics studies show MPO reacts 2× faster with terephthalic acid due to reduced steric hindrance, yielding coatings with superior hardness (3H vs. 2H) and adhesion (ASTM D3359) .
Q. How are enantioselective syntheses of MPO derivatives achieved for chiral polymer applications?
Advanced Research Question
Iridium-catalyzed double crotylation of MPO using BIPHEP ligands generates C₂-symmetric stereoquintets (e.g., s,s,s,s-3a) with >86% enantiomeric excess (ee). Chiral GC and NOESY NMR validate configuration, enabling synthesis of optically active polyesters for biomedical devices. Contaminants like s,s,a,a-isomers are removed via fractional crystallization .
Q. What analytical techniques characterize MPO’s role in ionic copolyesters?
Basic Research Question
Phosphonium-containing MPO copolymers (e.g., PBxPPyS) are analyzed via ¹H/³¹P NMR for composition, dynamic mechanical analysis (DMA) for Tg (−40 to 60°C), and tensile testing for modulus (0.1–1.2 GPa). Ionic interactions are quantified via conductivity measurements (10⁻⁶–10⁻⁴ S/cm) and XPS to confirm counterion distribution .
Properties
IUPAC Name |
2-methylpropane-1,3-diol | |
---|---|---|
Source | PubChem | |
URL | https://pubchem.ncbi.nlm.nih.gov | |
Description | Data deposited in or computed by PubChem | |
InChI |
InChI=1S/C4H10O2/c1-4(2-5)3-6/h4-6H,2-3H2,1H3 | |
Source | PubChem | |
URL | https://pubchem.ncbi.nlm.nih.gov | |
Description | Data deposited in or computed by PubChem | |
InChI Key |
QWGRWMMWNDWRQN-UHFFFAOYSA-N | |
Source | PubChem | |
URL | https://pubchem.ncbi.nlm.nih.gov | |
Description | Data deposited in or computed by PubChem | |
Canonical SMILES |
CC(CO)CO | |
Source | PubChem | |
URL | https://pubchem.ncbi.nlm.nih.gov | |
Description | Data deposited in or computed by PubChem | |
Molecular Formula |
C4H10O2 | |
Source | PubChem | |
URL | https://pubchem.ncbi.nlm.nih.gov | |
Description | Data deposited in or computed by PubChem | |
DSSTOX Substance ID |
DTXSID3029231 | |
Record name | 2-Methylpropane-1,3-diol | |
Source | EPA DSSTox | |
URL | https://comptox.epa.gov/dashboard/DTXSID3029231 | |
Description | DSSTox provides a high quality public chemistry resource for supporting improved predictive toxicology. | |
Molecular Weight |
90.12 g/mol | |
Source | PubChem | |
URL | https://pubchem.ncbi.nlm.nih.gov | |
Description | Data deposited in or computed by PubChem | |
Physical Description |
Liquid, Colorless liquid; [HSDB] Very faintly yellow-green liquid; [MSDSonline] | |
Record name | 1,3-Propanediol, 2-methyl- | |
Source | EPA Chemicals under the TSCA | |
URL | https://www.epa.gov/chemicals-under-tsca | |
Description | EPA Chemicals under the Toxic Substances Control Act (TSCA) collection contains information on chemicals and their regulations under TSCA, including non-confidential content from the TSCA Chemical Substance Inventory and Chemical Data Reporting. | |
Record name | 2-Methyl-1,3-propanediol | |
Source | Haz-Map, Information on Hazardous Chemicals and Occupational Diseases | |
URL | https://haz-map.com/Agents/3123 | |
Description | Haz-Map® is an occupational health database designed for health and safety professionals and for consumers seeking information about the adverse effects of workplace exposures to chemical and biological agents. | |
Explanation | Copyright (c) 2022 Haz-Map(R). All rights reserved. Unless otherwise indicated, all materials from Haz-Map are copyrighted by Haz-Map(R). No part of these materials, either text or image may be used for any purpose other than for personal use. Therefore, reproduction, modification, storage in a retrieval system or retransmission, in any form or by any means, electronic, mechanical or otherwise, for reasons other than personal use, is strictly prohibited without prior written permission. | |
Boiling Point |
211 °C | |
Record name | 2-Methyl-1,3-propanediol | |
Source | Hazardous Substances Data Bank (HSDB) | |
URL | https://pubchem.ncbi.nlm.nih.gov/source/hsdb/7267 | |
Description | The Hazardous Substances Data Bank (HSDB) is a toxicology database that focuses on the toxicology of potentially hazardous chemicals. It provides information on human exposure, industrial hygiene, emergency handling procedures, environmental fate, regulatory requirements, nanomaterials, and related areas. The information in HSDB has been assessed by a Scientific Review Panel. | |
Flash Point |
127 °C, 127 °C (261 °F) - closed cup | |
Record name | 2-Methyl-1,3-propanediol | |
Source | Haz-Map, Information on Hazardous Chemicals and Occupational Diseases | |
URL | https://haz-map.com/Agents/3123 | |
Description | Haz-Map® is an occupational health database designed for health and safety professionals and for consumers seeking information about the adverse effects of workplace exposures to chemical and biological agents. | |
Explanation | Copyright (c) 2022 Haz-Map(R). All rights reserved. Unless otherwise indicated, all materials from Haz-Map are copyrighted by Haz-Map(R). No part of these materials, either text or image may be used for any purpose other than for personal use. Therefore, reproduction, modification, storage in a retrieval system or retransmission, in any form or by any means, electronic, mechanical or otherwise, for reasons other than personal use, is strictly prohibited without prior written permission. | |
Record name | 2-Methyl-1,3-propanediol | |
Source | Hazardous Substances Data Bank (HSDB) | |
URL | https://pubchem.ncbi.nlm.nih.gov/source/hsdb/7267 | |
Description | The Hazardous Substances Data Bank (HSDB) is a toxicology database that focuses on the toxicology of potentially hazardous chemicals. It provides information on human exposure, industrial hygiene, emergency handling procedures, environmental fate, regulatory requirements, nanomaterials, and related areas. The information in HSDB has been assessed by a Scientific Review Panel. | |
Solubility |
In water, greater than or equal to 3000 mg/L at 25 °C | |
Record name | 2-Methyl-1,3-propanediol | |
Source | Hazardous Substances Data Bank (HSDB) | |
URL | https://pubchem.ncbi.nlm.nih.gov/source/hsdb/7267 | |
Description | The Hazardous Substances Data Bank (HSDB) is a toxicology database that focuses on the toxicology of potentially hazardous chemicals. It provides information on human exposure, industrial hygiene, emergency handling procedures, environmental fate, regulatory requirements, nanomaterials, and related areas. The information in HSDB has been assessed by a Scientific Review Panel. | |
Density |
1.015 g/ cu cm at 25 °C | |
Record name | 2-Methyl-1,3-propanediol | |
Source | Hazardous Substances Data Bank (HSDB) | |
URL | https://pubchem.ncbi.nlm.nih.gov/source/hsdb/7267 | |
Description | The Hazardous Substances Data Bank (HSDB) is a toxicology database that focuses on the toxicology of potentially hazardous chemicals. It provides information on human exposure, industrial hygiene, emergency handling procedures, environmental fate, regulatory requirements, nanomaterials, and related areas. The information in HSDB has been assessed by a Scientific Review Panel. | |
Vapor Density |
3.11 | |
Record name | 2-Methyl-1,3-propanediol | |
Source | Hazardous Substances Data Bank (HSDB) | |
URL | https://pubchem.ncbi.nlm.nih.gov/source/hsdb/7267 | |
Description | The Hazardous Substances Data Bank (HSDB) is a toxicology database that focuses on the toxicology of potentially hazardous chemicals. It provides information on human exposure, industrial hygiene, emergency handling procedures, environmental fate, regulatory requirements, nanomaterials, and related areas. The information in HSDB has been assessed by a Scientific Review Panel. | |
Vapor Pressure |
0.02 [mmHg], 2.1X10-2 mm Hg at 25 °C | |
Record name | 2-Methyl-1,3-propanediol | |
Source | Haz-Map, Information on Hazardous Chemicals and Occupational Diseases | |
URL | https://haz-map.com/Agents/3123 | |
Description | Haz-Map® is an occupational health database designed for health and safety professionals and for consumers seeking information about the adverse effects of workplace exposures to chemical and biological agents. | |
Explanation | Copyright (c) 2022 Haz-Map(R). All rights reserved. Unless otherwise indicated, all materials from Haz-Map are copyrighted by Haz-Map(R). No part of these materials, either text or image may be used for any purpose other than for personal use. Therefore, reproduction, modification, storage in a retrieval system or retransmission, in any form or by any means, electronic, mechanical or otherwise, for reasons other than personal use, is strictly prohibited without prior written permission. | |
Record name | 2-Methyl-1,3-propanediol | |
Source | Hazardous Substances Data Bank (HSDB) | |
URL | https://pubchem.ncbi.nlm.nih.gov/source/hsdb/7267 | |
Description | The Hazardous Substances Data Bank (HSDB) is a toxicology database that focuses on the toxicology of potentially hazardous chemicals. It provides information on human exposure, industrial hygiene, emergency handling procedures, environmental fate, regulatory requirements, nanomaterials, and related areas. The information in HSDB has been assessed by a Scientific Review Panel. | |
Color/Form |
Liquid, Clear, colorless liquid | |
CAS No. |
2163-42-0 | |
Record name | 2-Methyl-1,3-propanediol | |
Source | CAS Common Chemistry | |
URL | https://commonchemistry.cas.org/detail?cas_rn=2163-42-0 | |
Description | CAS Common Chemistry is an open community resource for accessing chemical information. Nearly 500,000 chemical substances from CAS REGISTRY cover areas of community interest, including common and frequently regulated chemicals, and those relevant to high school and undergraduate chemistry classes. This chemical information, curated by our expert scientists, is provided in alignment with our mission as a division of the American Chemical Society. | |
Explanation | The data from CAS Common Chemistry is provided under a CC-BY-NC 4.0 license, unless otherwise stated. | |
Record name | Methylpropanediol | |
Source | ChemIDplus | |
URL | https://pubchem.ncbi.nlm.nih.gov/substance/?source=chemidplus&sourceid=0002163420 | |
Description | ChemIDplus is a free, web search system that provides access to the structure and nomenclature authority files used for the identification of chemical substances cited in National Library of Medicine (NLM) databases, including the TOXNET system. | |
Record name | 1,3-Propanediol, 2-methyl- | |
Source | EPA Chemicals under the TSCA | |
URL | https://www.epa.gov/chemicals-under-tsca | |
Description | EPA Chemicals under the Toxic Substances Control Act (TSCA) collection contains information on chemicals and their regulations under TSCA, including non-confidential content from the TSCA Chemical Substance Inventory and Chemical Data Reporting. | |
Record name | 2-Methylpropane-1,3-diol | |
Source | EPA DSSTox | |
URL | https://comptox.epa.gov/dashboard/DTXSID3029231 | |
Description | DSSTox provides a high quality public chemistry resource for supporting improved predictive toxicology. | |
Record name | 1,3-Propanediol, 2-methyl | |
Source | European Chemicals Agency (ECHA) | |
URL | https://echa.europa.eu/substance-information/-/substanceinfo/100.106.510 | |
Description | The European Chemicals Agency (ECHA) is an agency of the European Union which is the driving force among regulatory authorities in implementing the EU's groundbreaking chemicals legislation for the benefit of human health and the environment as well as for innovation and competitiveness. | |
Explanation | Use of the information, documents and data from the ECHA website is subject to the terms and conditions of this Legal Notice, and subject to other binding limitations provided for under applicable law, the information, documents and data made available on the ECHA website may be reproduced, distributed and/or used, totally or in part, for non-commercial purposes provided that ECHA is acknowledged as the source: "Source: European Chemicals Agency, http://echa.europa.eu/". Such acknowledgement must be included in each copy of the material. ECHA permits and encourages organisations and individuals to create links to the ECHA website under the following cumulative conditions: Links can only be made to webpages that provide a link to the Legal Notice page. | |
Record name | METHYLPROPANEDIOL | |
Source | FDA Global Substance Registration System (GSRS) | |
URL | https://gsrs.ncats.nih.gov/ginas/app/beta/substances/N8F53B3R4R | |
Description | The FDA Global Substance Registration System (GSRS) enables the efficient and accurate exchange of information on what substances are in regulated products. Instead of relying on names, which vary across regulatory domains, countries, and regions, the GSRS knowledge base makes it possible for substances to be defined by standardized, scientific descriptions. | |
Explanation | Unless otherwise noted, the contents of the FDA website (www.fda.gov), both text and graphics, are not copyrighted. They are in the public domain and may be republished, reprinted and otherwise used freely by anyone without the need to obtain permission from FDA. Credit to the U.S. Food and Drug Administration as the source is appreciated but not required. | |
Record name | 2-Methyl-1,3-propanediol | |
Source | Hazardous Substances Data Bank (HSDB) | |
URL | https://pubchem.ncbi.nlm.nih.gov/source/hsdb/7267 | |
Description | The Hazardous Substances Data Bank (HSDB) is a toxicology database that focuses on the toxicology of potentially hazardous chemicals. It provides information on human exposure, industrial hygiene, emergency handling procedures, environmental fate, regulatory requirements, nanomaterials, and related areas. The information in HSDB has been assessed by a Scientific Review Panel. | |
Melting Point |
-91 °C | |
Record name | 2-Methyl-1,3-propanediol | |
Source | Hazardous Substances Data Bank (HSDB) | |
URL | https://pubchem.ncbi.nlm.nih.gov/source/hsdb/7267 | |
Description | The Hazardous Substances Data Bank (HSDB) is a toxicology database that focuses on the toxicology of potentially hazardous chemicals. It provides information on human exposure, industrial hygiene, emergency handling procedures, environmental fate, regulatory requirements, nanomaterials, and related areas. The information in HSDB has been assessed by a Scientific Review Panel. | |
Synthesis routes and methods I
Procedure details
Synthesis routes and methods II
Procedure details
Synthesis routes and methods III
Procedure details
Synthesis routes and methods IV
Procedure details
Synthesis routes and methods V
Procedure details
Retrosynthesis Analysis
AI-Powered Synthesis Planning: Our tool employs the Template_relevance Pistachio, Template_relevance Bkms_metabolic, Template_relevance Pistachio_ringbreaker, Template_relevance Reaxys, Template_relevance Reaxys_biocatalysis model, leveraging a vast database of chemical reactions to predict feasible synthetic routes.
One-Step Synthesis Focus: Specifically designed for one-step synthesis, it provides concise and direct routes for your target compounds, streamlining the synthesis process.
Accurate Predictions: Utilizing the extensive PISTACHIO, BKMS_METABOLIC, PISTACHIO_RINGBREAKER, REAXYS, REAXYS_BIOCATALYSIS database, our tool offers high-accuracy predictions, reflecting the latest in chemical research and data.
Strategy Settings
Precursor scoring | Relevance Heuristic |
---|---|
Min. plausibility | 0.01 |
Model | Template_relevance |
Template Set | Pistachio/Bkms_metabolic/Pistachio_ringbreaker/Reaxys/Reaxys_biocatalysis |
Top-N result to add to graph | 6 |
Feasible Synthetic Routes
Disclaimer and Information on In-Vitro Research Products
Please be aware that all articles and product information presented on BenchChem are intended solely for informational purposes. The products available for purchase on BenchChem are specifically designed for in-vitro studies, which are conducted outside of living organisms. In-vitro studies, derived from the Latin term "in glass," involve experiments performed in controlled laboratory settings using cells or tissues. It is important to note that these products are not categorized as medicines or drugs, and they have not received approval from the FDA for the prevention, treatment, or cure of any medical condition, ailment, or disease. We must emphasize that any form of bodily introduction of these products into humans or animals is strictly prohibited by law. It is essential to adhere to these guidelines to ensure compliance with legal and ethical standards in research and experimentation.