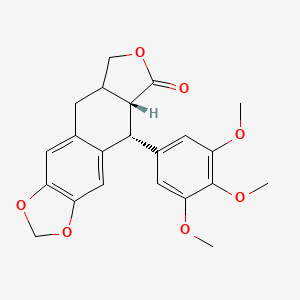
Isodeoxypodophyllotoxin
Overview
Description
Isodeoxypodophyllotoxin is a naturally occurring aryltetralin lignan, primarily derived from the roots and rhizomes of Podophyllum species. This compound is structurally related to podophyllotoxin and has garnered significant attention due to its potent biological activities, including antiviral and anticancer properties .
Preparation Methods
Synthetic Routes and Reaction Conditions: The synthesis of isodeoxypodophyllotoxin involves a series of intricate steps. One notable method is the free-radical carbocyclization reaction, where the lactone portion of the podophyllotoxin framework is assembled. This is followed by an intramolecular Friedel-Crafts reaction to construct the target structure . Another approach involves a multi-enzyme cascade in Escherichia coli, converting matairesinol to deoxypodophyllotoxin with high yield .
Industrial Production Methods: Industrial production of this compound is still in its nascent stages. the reconstitution of biosynthetic reactions in microbial systems, such as Escherichia coli, represents a promising avenue for sustainable production .
Chemical Reactions Analysis
Types of Reactions: Isodeoxypodophyllotoxin undergoes various chemical reactions, including oxidation, reduction, and substitution.
Common Reagents and Conditions:
Oxidation: Ceric ammonium nitrate is used for oxidative cleavage of the carbon-tin bond in the synthesis process.
Reduction: Tributyltin hydride is employed in the radical cyclization process.
Substitution: Various Friedel-Crafts conditions are applied for intramolecular reactions.
Major Products: The primary product of these reactions is this compound itself, along with other tri- and tetracyclic compounds formed during the synthesis .
Scientific Research Applications
Isodeoxypodophyllotoxin has a wide range of applications in scientific research:
Mechanism of Action
Isodeoxypodophyllotoxin exerts its effects primarily by interfering with the association between α-tubulin and β-tubulin, disrupting microtubule assembly and leading to cell cycle arrest in the G2/M phase . Its glycoside derivatives, such as etoposide and teniposide, exhibit potent anticancer activity by inserting into the type II topoisomerase-mediated DNA cleavage site, interrupting the catalytic cycle and causing DNA double-strand breaks .
Comparison with Similar Compounds
Podophyllotoxin: A closely related compound with similar antiviral and anticancer properties.
Deoxypodophyllotoxin: Another derivative with potent biological activities.
Epipodophyllotoxin: A precursor to chemotherapeutic agents like etoposide and teniposide.
Uniqueness: Isodeoxypodophyllotoxin is unique due to its specific stereochemistry and the distinct biological activities it exhibits compared to its analogs . Its synthesis often results in different diastereomers, each with varying bioactivities .
Properties
IUPAC Name |
(5R,5aR)-5-(3,4,5-trimethoxyphenyl)-5a,8,8a,9-tetrahydro-5H-[2]benzofuro[5,6-f][1,3]benzodioxol-6-one | |
---|---|---|
Source | PubChem | |
URL | https://pubchem.ncbi.nlm.nih.gov | |
Description | Data deposited in or computed by PubChem | |
InChI |
InChI=1S/C22H22O7/c1-24-17-6-12(7-18(25-2)21(17)26-3)19-14-8-16-15(28-10-29-16)5-11(14)4-13-9-27-22(23)20(13)19/h5-8,13,19-20H,4,9-10H2,1-3H3/t13?,19-,20+/m1/s1 | |
Source | PubChem | |
URL | https://pubchem.ncbi.nlm.nih.gov | |
Description | Data deposited in or computed by PubChem | |
InChI Key |
ZGLXUQQMLLIKAN-CFNCIARGSA-N | |
Source | PubChem | |
URL | https://pubchem.ncbi.nlm.nih.gov | |
Description | Data deposited in or computed by PubChem | |
Canonical SMILES |
COC1=CC(=CC(=C1OC)OC)C2C3C(CC4=CC5=C(C=C24)OCO5)COC3=O | |
Source | PubChem | |
URL | https://pubchem.ncbi.nlm.nih.gov | |
Description | Data deposited in or computed by PubChem | |
Isomeric SMILES |
COC1=CC(=CC(=C1OC)OC)[C@H]2[C@@H]3C(CC4=CC5=C(C=C24)OCO5)COC3=O | |
Source | PubChem | |
URL | https://pubchem.ncbi.nlm.nih.gov | |
Description | Data deposited in or computed by PubChem | |
Molecular Formula |
C22H22O7 | |
Source | PubChem | |
URL | https://pubchem.ncbi.nlm.nih.gov | |
Description | Data deposited in or computed by PubChem | |
Molecular Weight |
398.4 g/mol | |
Source | PubChem | |
URL | https://pubchem.ncbi.nlm.nih.gov | |
Description | Data deposited in or computed by PubChem | |
CAS No. |
69222-20-4 | |
Record name | Deoxypodophyllotoxin | |
Source | ChemIDplus | |
URL | https://pubchem.ncbi.nlm.nih.gov/substance/?source=chemidplus&sourceid=0069222204 | |
Description | ChemIDplus is a free, web search system that provides access to the structure and nomenclature authority files used for the identification of chemical substances cited in National Library of Medicine (NLM) databases, including the TOXNET system. | |
Retrosynthesis Analysis
AI-Powered Synthesis Planning: Our tool employs the Template_relevance Pistachio, Template_relevance Bkms_metabolic, Template_relevance Pistachio_ringbreaker, Template_relevance Reaxys, Template_relevance Reaxys_biocatalysis model, leveraging a vast database of chemical reactions to predict feasible synthetic routes.
One-Step Synthesis Focus: Specifically designed for one-step synthesis, it provides concise and direct routes for your target compounds, streamlining the synthesis process.
Accurate Predictions: Utilizing the extensive PISTACHIO, BKMS_METABOLIC, PISTACHIO_RINGBREAKER, REAXYS, REAXYS_BIOCATALYSIS database, our tool offers high-accuracy predictions, reflecting the latest in chemical research and data.
Strategy Settings
Precursor scoring | Relevance Heuristic |
---|---|
Min. plausibility | 0.01 |
Model | Template_relevance |
Template Set | Pistachio/Bkms_metabolic/Pistachio_ringbreaker/Reaxys/Reaxys_biocatalysis |
Top-N result to add to graph | 6 |
Feasible Synthetic Routes
Disclaimer and Information on In-Vitro Research Products
Please be aware that all articles and product information presented on BenchChem are intended solely for informational purposes. The products available for purchase on BenchChem are specifically designed for in-vitro studies, which are conducted outside of living organisms. In-vitro studies, derived from the Latin term "in glass," involve experiments performed in controlled laboratory settings using cells or tissues. It is important to note that these products are not categorized as medicines or drugs, and they have not received approval from the FDA for the prevention, treatment, or cure of any medical condition, ailment, or disease. We must emphasize that any form of bodily introduction of these products into humans or animals is strictly prohibited by law. It is essential to adhere to these guidelines to ensure compliance with legal and ethical standards in research and experimentation.