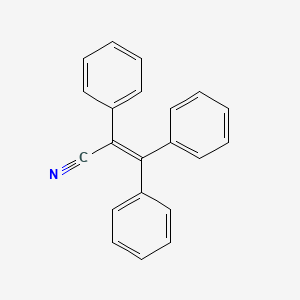
Triphenylacrylonitrile
Overview
Description
2,3,3-Triphenyl-acrylonitrile is an organic compound with the molecular formula C21H15NThis compound is characterized by its three phenyl groups attached to an acrylonitrile moiety, making it a highly aromatic and stable molecule .
Mechanism of Action
Target of Action
Triphenylacrylonitrile primarily targets the photophysical and photochemical processes of aggregation-induced emission luminogens (AIEgens) in distinct states . The compound’s primary role is to regulate these processes in a controllable manner .
Mode of Action
This compound interacts with its targets by altering the molecular conformation between highly twisted and more planar states . This interaction results in remarkable fluorescence redshifts . The compound also exhibits mechanochromic (MC) fluorescent behavior, which means it can change its photoluminescence colors or intensities in response to external mechanical stimuli .
Biochemical Pathways
The compound affects the biochemical pathways related to photophysical emission efficiency and color . It also influences photochemical photochromic and photoactivatable fluorescence behaviors . The introduction of heavy halogens like bromine into a this compound skeleton dramatically enhances the emission efficiency .
Result of Action
The molecular and cellular effects of this compound’s action are primarily observed in its emission properties. The compound exhibits aggregation-induced emission (AIE) behaviors . It shows remarkable fluorescence redshifts from sky-blue to light green upon fully grinding them . These effects are attributed to the compound’s phase transitions between crystalline and amorphous states .
Action Environment
Environmental factors significantly influence this compound’s action, efficacy, and stability. For instance, the compound’s mechanochromic properties are reversible upon the treatment of grinding and heating/fuming with DCM . The recovery time of the emission of the ground powders to that of crystals for certain derivatives of this compound was much shorter than others at the same temperature . This suggests that environmental temperature and mechanical stimuli can influence the compound’s photophysical properties.
Biochemical Analysis
Biochemical Properties
2,3,3-Triphenylacrylonitrile plays a significant role in biochemical reactions due to its ability to interact with various enzymes, proteins, and other biomolecules. It has been observed to participate in condensation reactions, forming β-enaminonitriles, which are crucial intermediates in the synthesis of pharmaceuticals, fungicides, dyes, and biological pesticides . The compound’s interaction with enzymes such as cytochrome P450 can lead to its metabolic transformation, affecting its biochemical activity and stability . Additionally, 2,3,3-Triphenylacrylonitrile can form complexes with proteins, influencing their structure and function, which is essential for understanding its role in biochemical pathways .
Cellular Effects
The effects of 2,3,3-Triphenylacrylonitrile on various cell types and cellular processes are profound. This compound has been shown to influence cell signaling pathways, gene expression, and cellular metabolism. For instance, it can modulate the activity of transcription factors, leading to changes in gene expression profiles . In cellular metabolism, 2,3,3-Triphenylacrylonitrile can affect the activity of metabolic enzymes, altering the flux of metabolites and impacting overall cellular function . These effects are crucial for understanding how this compound can be used in therapeutic applications and its potential cytotoxicity.
Molecular Mechanism
At the molecular level, 2,3,3-Triphenylacrylonitrile exerts its effects through various mechanisms. It can bind to specific biomolecules, such as enzymes and receptors, leading to inhibition or activation of their activity . For example, the compound’s interaction with cytochrome P450 enzymes can result in either the inhibition or enhancement of metabolic reactions . Additionally, 2,3,3-Triphenylacrylonitrile can influence gene expression by interacting with transcription factors and other regulatory proteins, thereby modulating the transcriptional activity of target genes .
Temporal Effects in Laboratory Settings
The stability and degradation of 2,3,3-Triphenylacrylonitrile over time in laboratory settings are critical for its application in biochemical research. Studies have shown that this compound is relatively stable under standard laboratory conditions, but it can undergo degradation when exposed to extreme temperatures or reactive chemicals . Long-term exposure to 2,3,3-Triphenylacrylonitrile has been observed to cause changes in cellular function, including alterations in cell viability and metabolic activity . These temporal effects are essential for designing experiments and interpreting results in biochemical studies.
Dosage Effects in Animal Models
The effects of 2,3,3-Triphenylacrylonitrile vary with different dosages in animal models. At low doses, the compound may exhibit minimal toxicity and can be used to study its therapeutic potential . At higher doses, 2,3,3-Triphenylacrylonitrile can cause adverse effects, including cytotoxicity and organ damage . These dosage-dependent effects are crucial for determining the safe and effective use of this compound in preclinical studies and potential therapeutic applications.
Metabolic Pathways
2,3,3-Triphenylacrylonitrile is involved in various metabolic pathways, interacting with enzymes and cofactors that facilitate its transformation and elimination . The compound can be metabolized by cytochrome P450 enzymes, leading to the formation of reactive intermediates that can further interact with cellular components . These metabolic pathways are essential for understanding the compound’s pharmacokinetics and potential toxicological effects.
Transport and Distribution
The transport and distribution of 2,3,3-Triphenylacrylonitrile within cells and tissues are influenced by its interaction with transporters and binding proteins . The compound can be actively transported across cell membranes, accumulating in specific cellular compartments . This distribution pattern is critical for understanding its cellular effects and potential therapeutic applications.
Subcellular Localization
2,3,3-Triphenylacrylonitrile exhibits specific subcellular localization, which can affect its activity and function. The compound can be directed to particular cellular compartments, such as the nucleus or mitochondria, through targeting signals or post-translational modifications . This localization is essential for its role in modulating cellular processes and its potential use in targeted therapies.
Preparation Methods
Synthetic Routes and Reaction Conditions: The synthesis of 2,3,3-Triphenyl-acrylonitrile typically involves the reaction of benzyl cyanide with benzophenone in the presence of sodium amide in liquid ammonia. The process is carried out in a three-necked round-bottomed flask fitted with a reflux condenser, mechanical stirrer, and an inlet tube. The reaction mixture is cooled using a Dry Ice and trichloroethylene bath, and the product is recrystallized from glacial acetic acid to obtain pure 2,3,3-Triphenyl-acrylonitrile .
Industrial Production Methods: Industrial production of 2,3,3-Triphenyl-acrylonitrile follows similar synthetic routes but on a larger scale. The use of continuous flow reactors and automated systems ensures consistent product quality and higher yields. Safety measures are crucial due to the use of hazardous reagents like sodium amide and liquid ammonia .
Chemical Reactions Analysis
Types of Reactions: 2,3,3-Triphenyl-acrylonitrile undergoes various chemical reactions, including:
Oxidation: The compound can be oxidized to form corresponding oxides.
Reduction: Reduction reactions can convert it into amines or other reduced forms.
Substitution: Electrophilic and nucleophilic substitution reactions can occur on the phenyl rings or the nitrile group.
Common Reagents and Conditions:
Oxidation: Common oxidizing agents include potassium permanganate and chromium trioxide.
Reduction: Reducing agents like lithium aluminum hydride or hydrogen in the presence of a catalyst are used.
Substitution: Reagents such as halogens, alkylating agents, and nucleophiles are employed under various conditions.
Major Products:
Oxidation: Formation of carboxylic acids or ketones.
Reduction: Formation of primary or secondary amines.
Substitution: Formation of substituted phenyl derivatives.
Scientific Research Applications
2,3,3-Triphenyl-acrylonitrile has diverse applications in scientific research:
Chemistry: Used as a precursor in organic synthesis and as a reagent in various chemical reactions.
Biology: Studied for its potential biological activities and interactions with biomolecules.
Medicine: Investigated for its pharmacological properties and potential therapeutic applications.
Industry: Utilized in the production of polymers, dyes, and other industrial chemicals.
Comparison with Similar Compounds
- 2,3,3-Triphenyl-prop-2-enenitrile
- 2,3,3-Triphenyl-2-propenenitrile
- alpha,beta-Diphenylcinnamonitrile
Comparison: 2,3,3-Triphenyl-acrylonitrile is unique due to its specific arrangement of phenyl groups and the presence of a nitrile group. This structure imparts distinct chemical and physical properties, making it more stable and reactive under certain conditions compared to its analogs .
Properties
IUPAC Name |
2,3,3-triphenylprop-2-enenitrile | |
---|---|---|
Source | PubChem | |
URL | https://pubchem.ncbi.nlm.nih.gov | |
Description | Data deposited in or computed by PubChem | |
InChI |
InChI=1S/C21H15N/c22-16-20(17-10-4-1-5-11-17)21(18-12-6-2-7-13-18)19-14-8-3-9-15-19/h1-15H | |
Source | PubChem | |
URL | https://pubchem.ncbi.nlm.nih.gov | |
Description | Data deposited in or computed by PubChem | |
InChI Key |
OPACMJHTQWVSKW-UHFFFAOYSA-N | |
Source | PubChem | |
URL | https://pubchem.ncbi.nlm.nih.gov | |
Description | Data deposited in or computed by PubChem | |
Canonical SMILES |
C1=CC=C(C=C1)C(=C(C2=CC=CC=C2)C3=CC=CC=C3)C#N | |
Source | PubChem | |
URL | https://pubchem.ncbi.nlm.nih.gov | |
Description | Data deposited in or computed by PubChem | |
Molecular Formula |
C21H15N | |
Source | PubChem | |
URL | https://pubchem.ncbi.nlm.nih.gov | |
Description | Data deposited in or computed by PubChem | |
DSSTOX Substance ID |
DTXSID60212329 | |
Record name | 2,3,3-Triphenylacrylonitrile | |
Source | EPA DSSTox | |
URL | https://comptox.epa.gov/dashboard/DTXSID60212329 | |
Description | DSSTox provides a high quality public chemistry resource for supporting improved predictive toxicology. | |
Molecular Weight |
281.3 g/mol | |
Source | PubChem | |
URL | https://pubchem.ncbi.nlm.nih.gov | |
Description | Data deposited in or computed by PubChem | |
CAS No. |
6304-33-2 | |
Record name | α-(Diphenylmethylene)benzeneacetonitrile | |
Source | CAS Common Chemistry | |
URL | https://commonchemistry.cas.org/detail?cas_rn=6304-33-2 | |
Description | CAS Common Chemistry is an open community resource for accessing chemical information. Nearly 500,000 chemical substances from CAS REGISTRY cover areas of community interest, including common and frequently regulated chemicals, and those relevant to high school and undergraduate chemistry classes. This chemical information, curated by our expert scientists, is provided in alignment with our mission as a division of the American Chemical Society. | |
Explanation | The data from CAS Common Chemistry is provided under a CC-BY-NC 4.0 license, unless otherwise stated. | |
Record name | 2,3,3-Triphenylacrylonitrile | |
Source | ChemIDplus | |
URL | https://pubchem.ncbi.nlm.nih.gov/substance/?source=chemidplus&sourceid=0006304332 | |
Description | ChemIDplus is a free, web search system that provides access to the structure and nomenclature authority files used for the identification of chemical substances cited in National Library of Medicine (NLM) databases, including the TOXNET system. | |
Record name | Triphenylacrylonitrile | |
Source | DTP/NCI | |
URL | https://dtp.cancer.gov/dtpstandard/servlet/dwindex?searchtype=NSC&outputformat=html&searchlist=42900 | |
Description | The NCI Development Therapeutics Program (DTP) provides services and resources to the academic and private-sector research communities worldwide to facilitate the discovery and development of new cancer therapeutic agents. | |
Explanation | Unless otherwise indicated, all text within NCI products is free of copyright and may be reused without our permission. Credit the National Cancer Institute as the source. | |
Record name | 2,3,3-Triphenylacrylonitrile | |
Source | EPA DSSTox | |
URL | https://comptox.epa.gov/dashboard/DTXSID60212329 | |
Description | DSSTox provides a high quality public chemistry resource for supporting improved predictive toxicology. | |
Record name | Triphenylacrylonitrile | |
Source | European Chemicals Agency (ECHA) | |
URL | https://echa.europa.eu/substance-information/-/substanceinfo/100.026.010 | |
Description | The European Chemicals Agency (ECHA) is an agency of the European Union which is the driving force among regulatory authorities in implementing the EU's groundbreaking chemicals legislation for the benefit of human health and the environment as well as for innovation and competitiveness. | |
Explanation | Use of the information, documents and data from the ECHA website is subject to the terms and conditions of this Legal Notice, and subject to other binding limitations provided for under applicable law, the information, documents and data made available on the ECHA website may be reproduced, distributed and/or used, totally or in part, for non-commercial purposes provided that ECHA is acknowledged as the source: "Source: European Chemicals Agency, http://echa.europa.eu/". Such acknowledgement must be included in each copy of the material. ECHA permits and encourages organisations and individuals to create links to the ECHA website under the following cumulative conditions: Links can only be made to webpages that provide a link to the Legal Notice page. | |
Record name | 2,3,3-Triphenylacrylonitrile | |
Source | FDA Global Substance Registration System (GSRS) | |
URL | https://gsrs.ncats.nih.gov/ginas/app/beta/substances/33Q5RZG6CP | |
Description | The FDA Global Substance Registration System (GSRS) enables the efficient and accurate exchange of information on what substances are in regulated products. Instead of relying on names, which vary across regulatory domains, countries, and regions, the GSRS knowledge base makes it possible for substances to be defined by standardized, scientific descriptions. | |
Explanation | Unless otherwise noted, the contents of the FDA website (www.fda.gov), both text and graphics, are not copyrighted. They are in the public domain and may be republished, reprinted and otherwise used freely by anyone without the need to obtain permission from FDA. Credit to the U.S. Food and Drug Administration as the source is appreciated but not required. | |
Retrosynthesis Analysis
AI-Powered Synthesis Planning: Our tool employs the Template_relevance Pistachio, Template_relevance Bkms_metabolic, Template_relevance Pistachio_ringbreaker, Template_relevance Reaxys, Template_relevance Reaxys_biocatalysis model, leveraging a vast database of chemical reactions to predict feasible synthetic routes.
One-Step Synthesis Focus: Specifically designed for one-step synthesis, it provides concise and direct routes for your target compounds, streamlining the synthesis process.
Accurate Predictions: Utilizing the extensive PISTACHIO, BKMS_METABOLIC, PISTACHIO_RINGBREAKER, REAXYS, REAXYS_BIOCATALYSIS database, our tool offers high-accuracy predictions, reflecting the latest in chemical research and data.
Strategy Settings
Precursor scoring | Relevance Heuristic |
---|---|
Min. plausibility | 0.01 |
Model | Template_relevance |
Template Set | Pistachio/Bkms_metabolic/Pistachio_ringbreaker/Reaxys/Reaxys_biocatalysis |
Top-N result to add to graph | 6 |
Feasible Synthetic Routes
ANone: 2,3,3-Triphenylacrylonitrile derivatives, often referred to as TPEs, can bind to the estrogen receptor (ER), competing with estradiol for binding sites. [] The specific interactions and binding affinity are influenced by the chemical substituents on the TPE molecule. For instance, hydroxylated TPEs have been shown to adopt a unique orientation within the ER binding site. [] The downstream effects of this interaction can vary depending on the specific TPE structure. Some TPEs exhibit estrogenic activity, stimulating the proliferation of ER-positive breast cancer cells like MCF7. [, , ] Others act as antiestrogens, inhibiting estradiol-promoted cell growth. [, ]
ANone: Yes, certain 2,3,3-Triphenylacrylonitrile derivatives have been shown to modulate protein kinase C (PKC) activity. [, , ] Their effect on PKC, either stimulatory or inhibitory, depends on factors like the specific TPE structure, enzyme activation conditions, and substrate used. For example, cytotoxicity in MCF7 cells has been correlated with the inhibition of diacylglycerol-stimulated PKC activity. []
ANone: The molecular formula of 2,3,3-Triphenylacrylonitrile is C21H15N, and its molecular weight is 281.35 g/mol.
ANone: Various spectroscopic techniques are used to characterize 2,3,3-Triphenylacrylonitrile and its derivatives. These include UV-Vis spectroscopy to study their absorption and emission properties, [, , , ] fluorescence spectroscopy to analyze their fluorescence behavior, [, , , ] and NMR spectroscopy for structural elucidation. [] Additionally, X-ray crystallography has been used to determine the single-crystal structures of several derivatives, providing insights into their molecular packing and intermolecular interactions. [, , , ]
ANone: Many 2,3,3-Triphenylacrylonitrile derivatives exhibit aggregation-induced emission (AIE). [, , , , , , , , , , ] This means they emit weakly or not at all in solution but become highly fluorescent in the aggregated state, such as in solid films or nanoparticles. This phenomenon is often attributed to the restriction of intramolecular motions (RIM) upon aggregation. [, , ] The type and degree of aggregation, influenced by factors like solvent and concentration, can significantly impact the emission color and intensity. [, , ]
ANone: The provided research papers do not focus on the catalytic properties of 2,3,3-Triphenylacrylonitrile. The studies primarily investigate its photophysical properties, structure-activity relationships, and potential applications in areas like OLEDs and sensors.
ANone: Computational methods, including DFT calculations and molecular modeling, provide valuable insights into the electronic structure, optical properties, and structure-activity relationships of 2,3,3-Triphenylacrylonitrile derivatives. [, , ] For example, DFT calculations have been used to explain the observed red-shifted absorption and emission in 2,3,3-Triphenylacrylonitrile-substituted BODIPYs compared to their tetraphenylethylene counterparts. [] QSAR studies, utilizing parameters like electronic structure, geometric structure, and topological indices, have been employed to predict the biological activity of 2,3,3-Triphenylacrylonitrile derivatives. [, , ] These studies aim to establish relationships between molecular structure and biological activity, facilitating the design of new compounds with improved properties. [, , ]
ANone: Modifying the chemical structure of 2,3,3-Triphenylacrylonitrile significantly impacts its interaction with the estrogen receptor and its subsequent biological activity. [, , ]
- Hydroxyl Substituents: Compounds with only hydroxy substituents tend to display more potent estrogenic activity, stimulating MCF7 cell proliferation. []
- Methylation: Methylation of hydroxyl groups can reduce estrogenic activity compared to their non-methylated counterparts. []- Bulky Side Chains: Introducing bulky hydrophobic or basic side chains, such as isopropyloxy or (diethylamino)ethoxy groups, can shift the activity profile towards antiestrogenic effects. [] These compounds tend to antagonize estradiol-promoted cell growth, and the extent of inhibition often correlates with the bulkiness of the side chain. []
ANone: Yes, QSAR studies suggest that the position of substituents on the phenyl rings significantly influences the binding affinity of 2,3,3-Triphenylacrylonitrile derivatives to the estrogen receptor. [] Specifically, electrophilic substitutions at specific positions on the A, B, and C rings of the molecule have been identified as crucial for ER binding affinity. []
Disclaimer and Information on In-Vitro Research Products
Please be aware that all articles and product information presented on BenchChem are intended solely for informational purposes. The products available for purchase on BenchChem are specifically designed for in-vitro studies, which are conducted outside of living organisms. In-vitro studies, derived from the Latin term "in glass," involve experiments performed in controlled laboratory settings using cells or tissues. It is important to note that these products are not categorized as medicines or drugs, and they have not received approval from the FDA for the prevention, treatment, or cure of any medical condition, ailment, or disease. We must emphasize that any form of bodily introduction of these products into humans or animals is strictly prohibited by law. It is essential to adhere to these guidelines to ensure compliance with legal and ethical standards in research and experimentation.