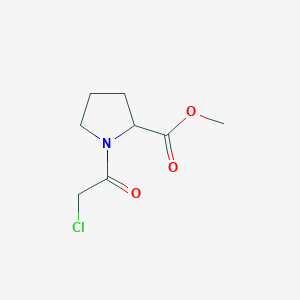
Vildagliptin Impurity 13
- Click on QUICK INQUIRY to receive a quote from our team of experts.
- With the quality product at a COMPETITIVE price, you can focus more on your research.
Overview
Description
Vildagliptin Impurity 13: is a specific impurity associated with the pharmaceutical compound Vildagliptin, which is used in the treatment of type 2 diabetes. Impurities in pharmaceutical compounds can arise from various sources, including the manufacturing process, degradation, or interactions with other substances. This compound is one of the many impurities that need to be identified, quantified, and controlled to ensure the safety and efficacy of the final pharmaceutical product.
Preparation Methods
Synthetic Routes and Reaction Conditions
The synthesis of Vildagliptin Impurity 13 typically involves multiple steps, starting from basic organic compounds. The synthetic route may include:
Starting Materials: The synthesis often begins with L-proline, a common starting material in organic synthesis.
Intermediate Formation: Through a series of reactions, including amide formation and cyclization, intermediates are formed.
Final Steps: The final steps involve specific conditions to introduce the impurity structure, such as controlled oxidation or reduction reactions.
Industrial Production Methods
In an industrial setting, the production of this compound involves large-scale synthesis with stringent control over reaction conditions to ensure consistency and purity. This includes:
Batch Processing: Large batches are processed under controlled temperatures and pressures.
Purification: Techniques such as crystallization, distillation, and chromatography are used to purify the impurity.
Quality Control: Rigorous testing is conducted to ensure the impurity meets regulatory standards.
Chemical Reactions Analysis
Types of Reactions
Vildagliptin Impurity 13 can undergo various chemical reactions, including:
Oxidation: This reaction involves the addition of oxygen or the removal of hydrogen, often using reagents like hydrogen peroxide or potassium permanganate.
Reduction: The removal of oxygen or addition of hydrogen, typically using reagents such as sodium borohydride or lithium aluminum hydride.
Substitution: Replacing one functional group with another, using reagents like halogens or nucleophiles.
Common Reagents and Conditions
Oxidizing Agents: Hydrogen peroxide, potassium permanganate.
Reducing Agents: Sodium borohydride, lithium aluminum hydride.
Solvents: Common solvents include methanol, ethanol, and dichloromethane.
Major Products Formed
The major products formed from these reactions depend on the specific conditions and reagents used. For example, oxidation may yield hydroxylated derivatives, while reduction could produce deoxygenated compounds.
Scientific Research Applications
Vildagliptin Impurity 13 has several applications in scientific research:
Pharmaceutical Research: Used as a reference standard in the development and quality control of Vildagliptin.
Analytical Chemistry: Employed in method validation and stability studies to ensure the purity of pharmaceutical products.
Toxicology Studies: Helps in assessing the genotoxic potential of impurities in pharmaceuticals.
Biological Research: Investigated for its biological activity and potential effects on metabolic pathways.
Mechanism of Action
The mechanism of action of Vildagliptin Impurity 13 is closely related to its parent compound, Vildagliptin. It may interact with similar molecular targets and pathways, including:
Dipeptidyl Peptidase-4 (DPP-4) Inhibition: Like Vildagliptin, the impurity may inhibit DPP-4, an enzyme involved in glucose metabolism.
Metabolic Pathways: It may affect various metabolic pathways, influencing glucose homeostasis and insulin secretion.
Comparison with Similar Compounds
Similar Compounds
Vildagliptin Impurity A: Another impurity with a similar structure but different functional groups.
Vildagliptin N-Oxide: An oxidized form of Vildagliptin with distinct chemical properties.
Vildagliptin D3: A deuterated form of Vildagliptin used in analytical studies.
Uniqueness
Vildagliptin Impurity 13 is unique due to its specific chemical structure and the particular synthetic route used to produce it. Its distinct properties make it an important reference standard in pharmaceutical research and quality control.
Properties
Molecular Formula |
C8H12ClNO3 |
---|---|
Molecular Weight |
205.64 g/mol |
IUPAC Name |
methyl 1-(2-chloroacetyl)pyrrolidine-2-carboxylate |
InChI |
InChI=1S/C8H12ClNO3/c1-13-8(12)6-3-2-4-10(6)7(11)5-9/h6H,2-5H2,1H3 |
InChI Key |
YYUKRFPRBNDTQF-UHFFFAOYSA-N |
Canonical SMILES |
COC(=O)C1CCCN1C(=O)CCl |
Origin of Product |
United States |
Disclaimer and Information on In-Vitro Research Products
Please be aware that all articles and product information presented on BenchChem are intended solely for informational purposes. The products available for purchase on BenchChem are specifically designed for in-vitro studies, which are conducted outside of living organisms. In-vitro studies, derived from the Latin term "in glass," involve experiments performed in controlled laboratory settings using cells or tissues. It is important to note that these products are not categorized as medicines or drugs, and they have not received approval from the FDA for the prevention, treatment, or cure of any medical condition, ailment, or disease. We must emphasize that any form of bodily introduction of these products into humans or animals is strictly prohibited by law. It is essential to adhere to these guidelines to ensure compliance with legal and ethical standards in research and experimentation.