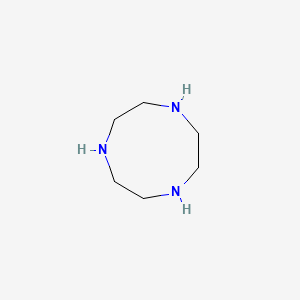
1,4,7-Triazacyclononane
Overview
Description
1,4,7-Triazacyclononane is a cyclic organic compound with the molecular formula C₆H₁₅N₃. It is a nine-membered ring containing three nitrogen atoms, which makes it a tridentate ligand. This compound is derived from cyclononane by replacing three equidistant methylene groups with nitrogen atoms. It is known for its ability to form stable complexes with various metal ions, making it a valuable ligand in coordination chemistry .
Mechanism of Action
Target of Action
1,4,7-Triazacyclononane primarily targets metalloids and transition metals . It is a popular tridentate ligand, meaning it can bind to one face of an octahedron of these metals . This compound is particularly effective in forming stable complexes with copper (II), a property that is greatly influenced by the number and type of substituents on the macrocyclic ring .
Mode of Action
The compound interacts with its targets by coordinating to metals in mid- and high oxidation states . The (this compound)M unit is kinetically inert, allowing further synthetic transformations on the other coordination sites . This interaction results in changes in the oxidation state of the metal .
Biochemical Pathways
This compound affects the biochemical pathways related to the activity of β-lactam antibiotics . It has been shown to inhibit metallo-β-lactamase (MBL), an enzyme that inactivates β-lactam antibiotics .
Result of Action
The primary result of this compound’s action is the restoration of the activity of β-lactam antibiotics against metallo-β-lactamase-producing Enterobacteriaceae . This is achieved by inhibiting the MBLs, thereby preventing the inactivation of β-lactam antibiotics .
Action Environment
The action, efficacy, and stability of this compound can be influenced by various environmental factors. For instance, the compound’s ability to form stable complexes with copper (II) can be affected by the presence of other metal ions . Additionally, the compound’s inhibitory activity against MBLs can be influenced by the presence of other enzymes or compounds in the environment .
Biochemical Analysis
Biochemical Properties
1,4,7-Triazacyclononane plays a significant role in biochemical reactions, particularly as a ligand for metal ions. It interacts with enzymes, proteins, and other biomolecules through its tridentate binding sites. For instance, it forms stable complexes with copper(II) ions, which are crucial for various enzymatic reactions . The compound’s ability to chelate metal ions enhances its utility in biochemical assays and therapeutic applications .
Cellular Effects
This compound has been shown to influence cellular processes by interacting with metalloenzymes and other metal-dependent proteins. It can restore the activity of β-lactam antibiotics against metallo-β-lactamase-producing bacteria, thereby combating antibiotic resistance . Additionally, studies have demonstrated that this compound does not induce cytotoxicity in human embryonic kidney cells, suggesting its potential for therapeutic use without adverse cellular effects .
Molecular Mechanism
At the molecular level, this compound exerts its effects by binding to the active sites of metalloenzymes, inhibiting their activity. This inhibition is achieved through the chelation of metal ions, which are essential for the enzymatic function . The compound’s tridentate binding mode allows it to form stable complexes with metal ions, thereby preventing the enzymatic degradation of antibiotics .
Temporal Effects in Laboratory Settings
In laboratory settings, the effects of this compound have been observed to change over time. The compound is stable under various conditions, but its activity can be influenced by factors such as pH and temperature . Long-term studies have shown that this compound maintains its efficacy in inhibiting metalloenzymes, with minimal degradation observed over extended periods .
Dosage Effects in Animal Models
The effects of this compound vary with different dosages in animal models. At lower doses, the compound effectively inhibits metalloenzymes without causing toxicity . At higher doses, some adverse effects, such as mild cytotoxicity, have been reported . These findings highlight the importance of optimizing dosage to achieve therapeutic benefits while minimizing potential risks.
Metabolic Pathways
This compound is involved in metabolic pathways that require metal ion chelation. It interacts with enzymes such as metallo-β-lactamases, inhibiting their activity and restoring the efficacy of β-lactam antibiotics . The compound’s ability to chelate metal ions also affects metabolic flux and metabolite levels, contributing to its therapeutic potential .
Transport and Distribution
Within cells and tissues, this compound is transported and distributed through interactions with metal ion transporters and binding proteins . These interactions facilitate the compound’s localization to specific cellular compartments, where it can exert its biochemical effects . The distribution of this compound is influenced by factors such as pH and the presence of competing metal ions .
Subcellular Localization
This compound is localized to various subcellular compartments, including the cytoplasm and organelles involved in metal ion metabolism . Its activity is modulated by targeting signals and post-translational modifications that direct it to specific cellular locations . These localization patterns are crucial for the compound’s function in inhibiting metalloenzymes and restoring antibiotic efficacy .
Preparation Methods
Synthetic Routes and Reaction Conditions
1,4,7-Triazacyclononane can be synthesized through several methods. One common approach involves the macrocyclization of diethylene triamine with ethylene glycol ditosylate. The reaction proceeds as follows :
- Diethylene triamine reacts with three equivalents of p-toluenesulfonyl chloride to form N,N’,N’'-tris(p-toluenesulfonyl)diethylene triamine.
- The intermediate is then treated with sodium ethoxide to form the corresponding sodium salt.
- The sodium salt undergoes macrocyclization with ethylene glycol ditosylate to yield 1,4,7-tris(p-toluenesulfonyl)-1,4,7-triazacyclononane.
- Finally, the tritosylated compound is deprotected using hydrobromic acid to obtain this compound.
Industrial Production Methods
Industrial production of this compound typically follows similar synthetic routes but on a larger scale. The use of continuous flow reactors and optimized reaction conditions can enhance the yield and purity of the final product .
Chemical Reactions Analysis
1,4,7-Triazacyclononane undergoes various chemical reactions, including:
Oxidation
Reagents and Conditions: Oxidizing agents such as hydrogen peroxide or potassium permanganate.
Major Products: Oxidized derivatives of this compound, often forming complexes with metal ions in higher oxidation states.
Reduction
Reagents and Conditions: Reducing agents like sodium borohydride or lithium aluminum hydride.
Major Products: Reduced forms of this compound, which can also form stable metal complexes.
Substitution
Reagents and Conditions: Alkyl halides or acyl chlorides in the presence of a base.
Major Products: Substituted derivatives of this compound, which can be further used in coordination chemistry.
Scientific Research Applications
1,4,7-Triazacyclononane has a wide range of applications in scientific research:
Chemistry
Coordination Chemistry: Used as a ligand to form stable complexes with transition metals, lanthanides, and actinides.
Catalysis: Employed in catalytic reactions, including oxidation and reduction processes.
Biology
Enzyme Inhibition: Acts as an inhibitor for metallo-β-lactamases, restoring the activity of β-lactam antibiotics against resistant bacteria.
Medicine
Radiotherapy: Chelates radiometals for use in targeted radiotherapy.
Drug Delivery: Forms complexes with therapeutic agents for targeted drug delivery.
Industry
Material Science: Incorporated into polymers and coatings to enhance their properties.
Environmental Science: Used in the removal of heavy metals from wastewater.
Comparison with Similar Compounds
1,4,7-Triazacyclononane is unique due to its tridentate binding mode and nine-membered ring structure. Similar compounds include:
1,4,7,10-Tetraazacyclododecane: A twelve-membered ring with four nitrogen atoms, offering tetradentate binding.
Piperazine: A six-membered ring with two nitrogen atoms, providing bidentate binding.
1,4,7-Trimethyl-1,4,7-triazacyclononane: A methylated derivative of this compound, which offers steric hindrance and different coordination properties.
Compared to these compounds, this compound provides a balance between ring size and nitrogen donor atoms, making it versatile for various applications in coordination chemistry and beyond .
Properties
IUPAC Name |
1,4,7-triazonane | |
---|---|---|
Source | PubChem | |
URL | https://pubchem.ncbi.nlm.nih.gov | |
Description | Data deposited in or computed by PubChem | |
InChI |
InChI=1S/C6H15N3/c1-2-8-5-6-9-4-3-7-1/h7-9H,1-6H2 | |
Source | PubChem | |
URL | https://pubchem.ncbi.nlm.nih.gov | |
Description | Data deposited in or computed by PubChem | |
InChI Key |
ITWBWJFEJCHKSN-UHFFFAOYSA-N | |
Source | PubChem | |
URL | https://pubchem.ncbi.nlm.nih.gov | |
Description | Data deposited in or computed by PubChem | |
Canonical SMILES |
C1CNCCNCCN1 | |
Source | PubChem | |
URL | https://pubchem.ncbi.nlm.nih.gov | |
Description | Data deposited in or computed by PubChem | |
Molecular Formula |
C6H15N3 | |
Source | PubChem | |
URL | https://pubchem.ncbi.nlm.nih.gov | |
Description | Data deposited in or computed by PubChem | |
DSSTOX Substance ID |
DTXSID50197095 | |
Record name | 1,4,7-Triazacyclononane | |
Source | EPA DSSTox | |
URL | https://comptox.epa.gov/dashboard/DTXSID50197095 | |
Description | DSSTox provides a high quality public chemistry resource for supporting improved predictive toxicology. | |
Molecular Weight |
129.20 g/mol | |
Source | PubChem | |
URL | https://pubchem.ncbi.nlm.nih.gov | |
Description | Data deposited in or computed by PubChem | |
Physical Description |
White crystals; [MSDSonline] | |
Record name | 1,4,7-Triazacyclononane | |
Source | Haz-Map, Information on Hazardous Chemicals and Occupational Diseases | |
URL | https://haz-map.com/Agents/2473 | |
Description | Haz-Map® is an occupational health database designed for health and safety professionals and for consumers seeking information about the adverse effects of workplace exposures to chemical and biological agents. | |
Explanation | Copyright (c) 2022 Haz-Map(R). All rights reserved. Unless otherwise indicated, all materials from Haz-Map are copyrighted by Haz-Map(R). No part of these materials, either text or image may be used for any purpose other than for personal use. Therefore, reproduction, modification, storage in a retrieval system or retransmission, in any form or by any means, electronic, mechanical or otherwise, for reasons other than personal use, is strictly prohibited without prior written permission. | |
CAS No. |
4730-54-5 | |
Record name | 1,4,7-Triazacyclononane | |
Source | CAS Common Chemistry | |
URL | https://commonchemistry.cas.org/detail?cas_rn=4730-54-5 | |
Description | CAS Common Chemistry is an open community resource for accessing chemical information. Nearly 500,000 chemical substances from CAS REGISTRY cover areas of community interest, including common and frequently regulated chemicals, and those relevant to high school and undergraduate chemistry classes. This chemical information, curated by our expert scientists, is provided in alignment with our mission as a division of the American Chemical Society. | |
Explanation | The data from CAS Common Chemistry is provided under a CC-BY-NC 4.0 license, unless otherwise stated. | |
Record name | 1,4,7-Triazacyclononane | |
Source | ChemIDplus | |
URL | https://pubchem.ncbi.nlm.nih.gov/substance/?source=chemidplus&sourceid=0004730545 | |
Description | ChemIDplus is a free, web search system that provides access to the structure and nomenclature authority files used for the identification of chemical substances cited in National Library of Medicine (NLM) databases, including the TOXNET system. | |
Record name | 1,4,7-Triazacyclononane | |
Source | EPA DSSTox | |
URL | https://comptox.epa.gov/dashboard/DTXSID50197095 | |
Description | DSSTox provides a high quality public chemistry resource for supporting improved predictive toxicology. | |
Record name | 1,4,7-Triazacyclononane | |
Source | European Chemicals Agency (ECHA) | |
URL | https://echa.europa.eu/information-on-chemicals | |
Description | The European Chemicals Agency (ECHA) is an agency of the European Union which is the driving force among regulatory authorities in implementing the EU's groundbreaking chemicals legislation for the benefit of human health and the environment as well as for innovation and competitiveness. | |
Explanation | Use of the information, documents and data from the ECHA website is subject to the terms and conditions of this Legal Notice, and subject to other binding limitations provided for under applicable law, the information, documents and data made available on the ECHA website may be reproduced, distributed and/or used, totally or in part, for non-commercial purposes provided that ECHA is acknowledged as the source: "Source: European Chemicals Agency, http://echa.europa.eu/". Such acknowledgement must be included in each copy of the material. ECHA permits and encourages organisations and individuals to create links to the ECHA website under the following cumulative conditions: Links can only be made to webpages that provide a link to the Legal Notice page. | |
Record name | 1,4,7-TRIAZACYCLONONANE | |
Source | FDA Global Substance Registration System (GSRS) | |
URL | https://gsrs.ncats.nih.gov/ginas/app/beta/substances/2UIF93C5H3 | |
Description | The FDA Global Substance Registration System (GSRS) enables the efficient and accurate exchange of information on what substances are in regulated products. Instead of relying on names, which vary across regulatory domains, countries, and regions, the GSRS knowledge base makes it possible for substances to be defined by standardized, scientific descriptions. | |
Explanation | Unless otherwise noted, the contents of the FDA website (www.fda.gov), both text and graphics, are not copyrighted. They are in the public domain and may be republished, reprinted and otherwise used freely by anyone without the need to obtain permission from FDA. Credit to the U.S. Food and Drug Administration as the source is appreciated but not required. | |
Synthesis routes and methods I
Procedure details
Synthesis routes and methods II
Procedure details
Retrosynthesis Analysis
AI-Powered Synthesis Planning: Our tool employs the Template_relevance Pistachio, Template_relevance Bkms_metabolic, Template_relevance Pistachio_ringbreaker, Template_relevance Reaxys, Template_relevance Reaxys_biocatalysis model, leveraging a vast database of chemical reactions to predict feasible synthetic routes.
One-Step Synthesis Focus: Specifically designed for one-step synthesis, it provides concise and direct routes for your target compounds, streamlining the synthesis process.
Accurate Predictions: Utilizing the extensive PISTACHIO, BKMS_METABOLIC, PISTACHIO_RINGBREAKER, REAXYS, REAXYS_BIOCATALYSIS database, our tool offers high-accuracy predictions, reflecting the latest in chemical research and data.
Strategy Settings
Precursor scoring | Relevance Heuristic |
---|---|
Min. plausibility | 0.01 |
Model | Template_relevance |
Template Set | Pistachio/Bkms_metabolic/Pistachio_ringbreaker/Reaxys/Reaxys_biocatalysis |
Top-N result to add to graph | 6 |
Feasible Synthetic Routes
Q1: What is the molecular formula and weight of 1,4,7-Triazacyclononane?
A1: The molecular formula of this compound is C6H15N3, and its molecular weight is 129.20 g/mol.
Q2: Are there any characteristic spectroscopic data for this compound?
A2: While the provided research papers do not delve into detailed spectroscopic characterization of the unsubstituted this compound itself, they extensively discuss spectroscopic data (including NMR, IR, EPR) of various metal complexes and derivatives.
Q3: What are the catalytic applications of this compound metal complexes?
A3: Metal complexes of this compound and its derivatives exhibit catalytic activity in various reactions, including:
- Oxidation Reactions: Mn, Fe, Co, and Ru complexes catalyze the oxidation of alkanes, alkenes, and alcohols using hydrogen peroxide as an oxidant. [, , ] Selectivity can be tuned by modifying the ligand structure, adding co-catalysts, and controlling reaction conditions.
- Phosphodiester Cleavage: Cu(II) complexes show promising activity in cleaving phosphodiester bonds in model substrates like bis(p-nitrophenyl)phosphate (BNPP) and DNA. [, ]
- DNA Hydrolysis: Trinuclear complexes of this compound derivatives demonstrate DNA hydrolysis activity, with varying cleavage patterns depending on the buffer system. []
Q4: How does modifying the structure of this compound impact its metal binding and catalytic properties?
A4: Introducing various substituents on the nitrogen atoms or the carbon backbone of this compound significantly influences its coordination chemistry and catalytic activity.
- Pendant Arms: Adding pendant arms with different donor groups (e.g., carboxylates, phosphinates, phenolates, alkoxides) alters the denticity and chelating ability of the ligand, affecting the stability and reactivity of the metal complexes. [, , , , , , , ] For example, incorporating phosphinate groups enhances the selectivity for Ga(III) over other metal ions. []
- Steric Effects: Bulky substituents on the nitrogen atoms can affect the accessibility of the metal center, influencing substrate binding and catalytic activity. []
- Chirality: Introducing chiral centers on the pendant arms can lead to diastereomeric complexes with distinct properties. [, ] For instance, chiral Mn(III)-Mn(IV) complexes exhibit enantioselectivity in the epoxidation of indene. []
Q5: What is known about the stability of this compound and its metal complexes?
A6: While the papers primarily focus on the thermodynamic stability of the metal complexes, they also demonstrate the kinetic inertness of some Ga(III) complexes in acidic and alkaline solutions. [] The stability of radiolabeled complexes like [Ga18F(Bn-NODP)] has been investigated for PET imaging applications, with the complex showing excellent stability in aqueous and PBS solutions. []
Q6: Are there any reported formulation strategies for improving the stability, solubility, or bioavailability of this compound derivatives?
A7: While the research papers don't provide specific formulation strategies, they highlight the use of different counterions (e.g., PF6–, ClO4–, BF4–) to improve the solubility and crystallization of metal complexes. [, , , , , ] Additionally, incorporating hydrophilic substituents on the pendant arms could enhance water solubility.
Q7: What is the role of computational chemistry in understanding this compound and its complexes?
A8: Molecular mechanics (MMX) calculations have been used to study the structure and Mg(II)/Ca(II) selectivity of this compound derivatives. [] Density functional theory (DFT) calculations provide insights into the electronic structure, stability, and reactivity of metal complexes. []
Q8: What are some potential applications of this compound and its derivatives beyond catalysis?
A8: The unique properties of this compound and its metal complexes make them attractive candidates for various applications:
- Magnetic Resonance Imaging (MRI): Mn(II) complexes exhibit favorable relaxometric properties, making them potentially useful as MRI contrast agents. []
- Positron Emission Tomography (PET): Ga(III) complexes, particularly those with high stability and fluoride binding affinity, show promise as molecular scaffolds for 18F binding in PET imaging. []
- Luminescence Imaging: Tb(III) complexes of this compound derivatives display high luminescence efficiency, making them suitable for time-resolved luminescence imaging. []
Disclaimer and Information on In-Vitro Research Products
Please be aware that all articles and product information presented on BenchChem are intended solely for informational purposes. The products available for purchase on BenchChem are specifically designed for in-vitro studies, which are conducted outside of living organisms. In-vitro studies, derived from the Latin term "in glass," involve experiments performed in controlled laboratory settings using cells or tissues. It is important to note that these products are not categorized as medicines or drugs, and they have not received approval from the FDA for the prevention, treatment, or cure of any medical condition, ailment, or disease. We must emphasize that any form of bodily introduction of these products into humans or animals is strictly prohibited by law. It is essential to adhere to these guidelines to ensure compliance with legal and ethical standards in research and experimentation.