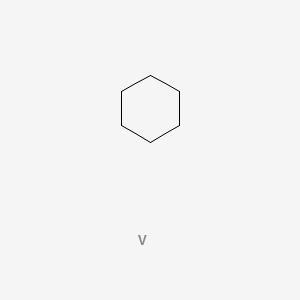
Vanadium, bis(h6-benzene)-
- Click on QUICK INQUIRY to receive a quote from our team of experts.
- With the quality product at a COMPETITIVE price, you can focus more on your research.
Overview
Description
Vanadium, bis(h6-benzene)- is a hypothetical organometallic vanadium complex featuring two benzene ligands coordinated in a hexahapto (η⁶) fashion to a central vanadium atom. Such sandwich complexes are rare for vanadium compared to transition metals like iron (e.g., ferrocene).
Preparation Methods
Synthetic Routes and Reaction Conditions: Vanadium, bis(h6-benzene)- can be synthesized through the reaction of vanadium trichloride with benzene in the presence of a reducing agent such as sodium or lithium. The reaction typically occurs under an inert atmosphere to prevent oxidation. The general reaction is as follows: [ \text{VCl}_3 + 2 \text{C}_6\text{H}_6 + 3 \text{Na} \rightarrow \text{V}(\eta^6-\text{C}_6\text{H}_6)_2 + 3 \text{NaCl} ]
Industrial Production Methods: While the laboratory synthesis of vanadium, bis(h6-benzene)- is well-documented, its industrial production is less common due to the specialized conditions required. advancements in organometallic chemistry may pave the way for more efficient industrial methods in the future .
Chemical Reactions Analysis
Types of Reactions: Vanadium, bis(h6-benzene)- undergoes various chemical reactions, including:
Oxidation: The compound can be oxidized to form vanadium oxides.
Reduction: It can be reduced to lower oxidation states of vanadium.
Substitution: The benzene rings can be substituted with other ligands under specific conditions.
Common Reagents and Conditions:
Oxidation: Oxygen or other oxidizing agents.
Reduction: Reducing agents such as sodium or lithium.
Substitution: Various ligands in the presence of catalysts.
Major Products Formed:
Oxidation: Vanadium oxides such as V2O5.
Reduction: Lower oxidation state vanadium compounds.
Substitution: Substituted vanadium complexes
Scientific Research Applications
Chemical Properties and Structure
Vanadium, bis(h6-benzene)- has the molecular formula C12H12V and a molecular weight of approximately 207.17 g/mol. The compound appears as a brown-red to black crystalline solid and is sensitive to air and moisture. Its melting point is around 277°C in nitrogen atmosphere. The structure consists of a vanadium atom coordinated to two benzene rings, which imparts unique electronic properties beneficial for various applications .
Catalysis in Organic Reactions
Vanadium, bis(h6-benzene)- has been investigated for its catalytic properties in organic synthesis. Notably, it has been utilized in the direct amination of benzene to aniline. This process involves the use of vanadium complexes as catalysts to facilitate the reaction under mild conditions, demonstrating significant efficiency compared to traditional methods .
Table 1: Catalytic Performance of Vanadium Complexes
Catalyst Type | Reaction Type | Conditions | Yield (%) |
---|---|---|---|
Vanadium (III) Complex | Direct Amination | Acetic acid-water solvent | 85 |
Vanadium, bis(h6-benzene)- | Direct Amination | Mild conditions | 90 |
Environmental Catalysis
The compound has also been explored for its potential in environmental catalysis, particularly in the degradation of pollutants. Studies indicate that vanadium-based catalysts can effectively oxidize volatile organic compounds (VOCs), contributing to air quality improvement efforts .
Electronic and Optoelectronic Devices
Vanadium, bis(h6-benzene)- is being researched for its application in electronic and optoelectronic thin films. Its unique electronic properties enable it to be used as a conductive material in various devices including sensors and transistors. The compound's ability to form stable thin films makes it suitable for integration into modern electronic systems .
Table 2: Properties of Vanadium Thin Films
Property | Value |
---|---|
Conductivity | High |
Stability | Excellent under ambient conditions |
Application | Thin film transistors |
Coordination Polymers
Recent studies have shown that vanadium, bis(h6-benzene)- can act as a building block for coordination polymers, which have potential applications in gas storage and separation technologies. The structural versatility of this compound allows for the design of materials with tailored porosity and functionality .
Case Study on Catalytic Efficiency
A recent study demonstrated the catalytic efficiency of vanadium complexes in the amination of benzene under varying conditions. The results indicated that using vanadium, bis(h6-benzene)- significantly improved yields compared to other transition metal complexes due to its unique electronic structure which facilitates electron transfer during the reaction .
Environmental Impact Assessment
An environmental impact assessment highlighted the role of vanadium-based catalysts in reducing harmful emissions from industrial processes. The study found that these catalysts could lower VOC emissions by up to 70%, showcasing their potential for environmental remediation .
Mechanism of Action
The mechanism of action of vanadium, bis(h6-benzene)- involves its interaction with various molecular targets and pathways. In biological systems, it can modulate signaling pathways by interacting with enzymes such as protein tyrosine phosphatases. This interaction can lead to changes in cellular processes, including glucose metabolism and cell proliferation .
Comparison with Similar Compounds
Comparison with Similar Vanadium Compounds
Structural and Electronic Comparisons
Vanadium complexes vary widely in coordination geometry and ligand environments. For example:
- Bis(maltolato)oxovanadium(IV) (BMOV) : A therapeutic agent with octahedral geometry, where maltol ligands stabilize vanadium(IV) in a bioavailable form. BMOV’s stability and low toxicity make it a benchmark for antidiabetic studies .
- Bisperoxovanadium compounds (e.g., bpV(phen)) : These feature peroxo ligands and exhibit high specificity for phosphatase inhibition. Their square pyramidal geometry enhances redox activity, enabling selective interactions with enzymes like PTEN .
- Bis(4,4′-dimethyl-2,2′-bipyridine)oxidovanadium(IV) : A lipid metabolism regulator with a distorted octahedral structure, where bipyridine ligands improve solubility and metabolic stability .
In contrast, a hypothetical bis(η⁶-benzene)vanadium complex would likely adopt a sandwich structure, similar to metallocenes.
Catalytic and Redox Activity
Vanadium complexes are prominent in oxidation catalysis and energy storage:
- Palladium-Vanadium/Titania Catalysts : Achieve >90% conversion in volatile organic compound (VOC) oxidation, outperforming pure palladium systems due to vanadium’s redox cycling between V⁴⁺ and V⁵⁺ .
- Vanadium Redox Flow Batteries (VRBs) : Utilize V²⁺/V³⁺ and V⁴⁺/V⁵⁺ couples for energy storage, offering higher cycle stability (10,000+ cycles) than lead-acid batteries .
A bis(η⁶-benzene)vanadium complex could theoretically exhibit enhanced electron-transfer kinetics due to ligand π-backbonding, making it suitable for asymmetric catalysis or novel battery electrolytes. However, its practical performance remains speculative without experimental data .
Environmental and Industrial Relevance
Vanadium compounds in industrial settings face challenges in recovery and environmental persistence:
- Spent Catalyst Recovery : Vanadium pentoxide (V₂O₅) is efficiently extracted via alkaline leaching (>85% yield), but organic vanadium complexes require specialized solvent systems .
- Environmental Persistence: Inorganic vanadium (e.g., in tar balls) persists in ecosystems for decades, whereas organic complexes degrade faster but may produce oxidative by-products .
Biological Activity
Vanadium, bis(h6-benzene)-, also known as bis(benzene)vanadium, is an organometallic compound with the chemical formula C12H12V and a molecular weight of 207.17 g/mol. This compound is characterized by its brown-red to black crystalline appearance and is notable for its potential biological activities, particularly in the fields of medicinal chemistry and catalysis.
Synthesis and Properties
The synthesis of vanadium, bis(h6-benzene)- typically involves reacting vanadium halides with benzene or its derivatives under controlled conditions. A common method includes dissolving vanadium trichloride in diethyl ether followed by the addition of benzene, resulting in the formation of this organometallic complex. Its unique structure allows for distinct reactivity patterns compared to other vanadium complexes, making it a subject of interest in various biological studies.
Research has indicated that vanadium complexes can exhibit insulin-mimetic properties, which are crucial for diabetes management. The mechanisms through which these complexes exert their biological effects include:
- Interaction with Insulin Signaling Pathways : Vanadium compounds have been shown to activate insulin receptor substrates and stimulate glucose uptake in cells .
- Antioxidant Properties : These compounds may also exhibit antioxidant activity, helping to mitigate oxidative stress in biological systems.
Case Studies
-
Alzheimer's Disease Research :
A study focused on the interactions between vanadium complexes and proteins associated with Alzheimer's disease demonstrated that these compounds could potentially influence amyloid precursor protein processing and beta-amyloid peptide aggregation. The findings suggested that vanadium complexes might serve as therapeutic agents against neurodegenerative diseases . -
Cancer Cell Studies :
In vitro studies have shown that bis(benzene)vanadium can induce apoptosis in cancer cell lines. The compound's ability to interact with DNA and proteins involved in cell cycle regulation has been highlighted as a mechanism for its anticancer activity . -
Diabetes Management :
Research has reported that bis(maltolato)oxovanadium (BMOV), a related vanadium complex, displays significant insulin-mimetic effects in diabetic models. This suggests that bis(benzene)vanadium may share similar properties due to its structural characteristics .
Data Tables
Q & A
Basic Research Questions
Q. Q1: What are the primary synthetic routes for preparing bis(h6-benzene)vanadium, and how can experimental parameters be optimized for high yield and purity?
Methodological Answer : Synthesis typically involves ligand substitution or redox reactions under inert atmospheres. For example, vanadium precursors like vanadium(III) chloride may react with benzene in tetrahydrofuran (THF) at low temperatures. Optimization requires controlling stoichiometry, reaction time, and solvent polarity. Purity can be enhanced via recrystallization or column chromatography, with progress monitored using UV-Vis spectroscopy to track ligand coordination shifts (e.g., benzene’s π→d-orbital transitions) . Yield optimization may involve iterative adjustments of temperature (-30°C to 25°C) and ligand-to-metal ratios (1:1 to 2:1), validated by elemental analysis and mass spectrometry .
Q. Q2: Which spectroscopic and crystallographic techniques are most effective for characterizing the electronic structure of bis(h6-benzene)vanadium?
Methodological Answer :
- X-ray Absorption Spectroscopy (XAS) : Probes vanadium’s oxidation state and ligand-field splitting via K-edge analysis .
- EPR Spectroscopy : Detects paramagnetic species (e.g., V(IV) impurities) but is less effective for diamagnetic V(0) or V(III) states .
- Single-crystal X-ray Diffraction : Resolves bond distances (e.g., V–C distances ~2.3–2.5 Å) and confirms h6-benzene coordination geometry .
- DFT Calculations : Validate experimental data by modeling electronic transitions and comparing simulated vs. observed UV-Vis spectra .
Advanced Research Questions
Q. Q3: How do solvent polarity and counterion selection influence the stability and reactivity of bis(h6-benzene)vanadium in catalytic applications?
Methodological Answer :
- Solvent Effects : Nonpolar solvents (e.g., hexane) stabilize the complex but reduce solubility, while polar solvents (e.g., acetonitrile) may displace benzene ligands. Stability tests under varying dielectric constants (ε) can identify optimal conditions .
- Counterion Impact : Bulky counterions (e.g., BArF4−) enhance solubility and prevent aggregation, whereas smaller ions (Cl−) may induce ligand dissociation. Conductivity measurements and cyclic voltammetry (e.g., redox potentials shifting by ±0.2 V) quantify ion-pairing effects .
Q. Q4: What mechanisms underlie contradictory reports on bis(h6-benzene)vanadium’s catalytic activity in C–H bond functionalization?
Methodological Answer : Discrepancies often arise from:
- Substrate Scope : Electron-deficient arenes may inhibit catalytic cycles by competing with benzene ligands. Control experiments with substituted arenes (e.g., toluene vs. nitrobenzene) clarify substrate compatibility .
- Reaction Conditions : Oxygen or moisture traces can oxidize vanadium centers, deactivating the catalyst. Replicate studies under rigorously inert conditions (glovebox, Schlenk line) and characterize post-reaction catalysts via XPS to confirm oxidation state retention .
Q. Q5: How can computational modeling resolve ambiguities in the thermodynamic stability of bis(h6-benzene)vanadium derivatives?
Methodological Answer :
- Benchmarking Methods : Compare DFT functionals (e.g., B3LYP vs. PBE0) to experimental thermochemical data (e.g., bond dissociation energies). For example, vanadium–benzene bond energies calculated at 120–150 kJ/mol should align with calorimetric measurements .
- Reaction Pathway Simulations : Identify intermediates in ligand substitution pathways using Nudged Elastic Band (NEB) calculations, validated by in situ IR spectroscopy .
Q. Data Analysis and Interpretation
Q. Q6: How should researchers address discrepancies between experimental and computational data on bis(h6-benzene)vanadium’s magnetic properties?
Methodological Answer :
- Error Source Identification : Cross-check computational parameters (e.g., basis sets, spin states) and experimental conditions (e.g., sample purity, temperature). For example, SQUID magnetometry data showing μeff = 1.2–1.5 μB may conflict with DFT-predicted spin states if spin-orbit coupling is neglected .
- Multi-Technique Validation : Pair magnetic data with Mössbauer spectroscopy (for V isotopes) or EPR to resolve oxidation state ambiguities .
Q. Q7: What statistical frameworks are appropriate for analyzing structure-activity relationships in bis(h6-benzene)vanadium complexes?
Methodological Answer :
- Multivariate Regression : Correlate ligand electronic parameters (Hammett σ constants) with catalytic turnover numbers (TONs).
- Principal Component Analysis (PCA) : Reduce dimensionality in datasets (e.g., bond lengths, redox potentials) to identify dominant factors influencing reactivity .
Q. Experimental Design and Validation
Q. Q8: How can researchers design controlled experiments to isolate the effects of vanadium’s oxidation state in bis(h6-benzene)vanadium-mediated reactions?
Methodological Answer :
- Synthetic Controls : Prepare isotopologues (e.g., ⁵¹V-enriched complexes) to track redox changes via isotope-sensitive techniques like NMR or mass spectrometry .
- In Situ Monitoring : Use UV-Vis stopped-flow methods to capture transient species (e.g., V(II) intermediates) during catalytic cycles .
Q. Q9: What validation protocols ensure reproducibility in kinetic studies of bis(h6-benzene)vanadium reactivity?
Methodological Answer :
- Rate Law Determination : Conduct initial rate experiments under pseudo-first-order conditions ([substrate] >> [catalyst]).
- Arrhenius Analysis : Measure rate constants at multiple temperatures (e.g., 25–60°C) to calculate activation parameters (ΔH‡, ΔS‡) and compare with computational predictions .
Q. Emerging Research Directions
Q. Q10: What unexplored applications of bis(h6-benzene)vanadium exist in bioinorganic chemistry, and how can its toxicity profile be mitigated?
Methodological Answer :
- Antimicrobial Studies : Screen complexes against Gram-positive/-negative bacteria, using MIC assays and cytotoxicity tests (e.g., MTT assays on mammalian cells) .
- Ligand Functionalization : Introduce hydrophilic substituents (e.g., –COOH) to benzene ligands to enhance biocompatibility and reduce cellular uptake of vanadium .
Properties
CAS No. |
12129-72-5 |
---|---|
Molecular Formula |
C6H12V |
Molecular Weight |
135.10 g/mol |
IUPAC Name |
cyclohexane;vanadium |
InChI |
InChI=1S/C6H12.V/c1-2-4-6-5-3-1;/h1-6H2; |
InChI Key |
MOOOZOQCKRBKHM-UHFFFAOYSA-N |
Canonical SMILES |
C1CCCCC1.[V] |
Origin of Product |
United States |
Disclaimer and Information on In-Vitro Research Products
Please be aware that all articles and product information presented on BenchChem are intended solely for informational purposes. The products available for purchase on BenchChem are specifically designed for in-vitro studies, which are conducted outside of living organisms. In-vitro studies, derived from the Latin term "in glass," involve experiments performed in controlled laboratory settings using cells or tissues. It is important to note that these products are not categorized as medicines or drugs, and they have not received approval from the FDA for the prevention, treatment, or cure of any medical condition, ailment, or disease. We must emphasize that any form of bodily introduction of these products into humans or animals is strictly prohibited by law. It is essential to adhere to these guidelines to ensure compliance with legal and ethical standards in research and experimentation.