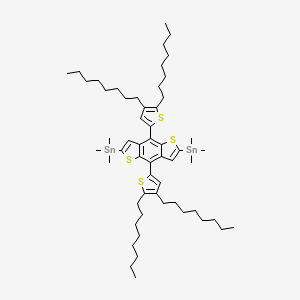
2,6-Bis(triMethyltin)-dioctyl-
- Click on QUICK INQUIRY to receive a quote from our team of experts.
- With the quality product at a COMPETITIVE price, you can focus more on your research.
Overview
Description
The compound 2,6-Bis(trimethyltin)-dioctyl- refers to a class of organotin derivatives featuring a central aromatic or heterocyclic core substituted with two trimethyltin (Sn(CH₃)₃) groups at the 2,6-positions and dioctyl (or dioctyloxy) substituents. A prominent example is 2,6-Bis(trimethyltin)-dithieno[3,2-b:2',3'-d]thiophene (CAS: 502764-57-0), which has a molecular formula of C₁₄H₂₀S₃Sn₂ and a molecular weight of 521.92 g/mol . This compound is widely utilized as a monomer in synthesizing conjugated polymers for organic photovoltaics (OPV) and organic thin-film transistors (OTFTs) . Its structure combines electron-rich dithieno[3,2-b:2',3'-d]thiophene (DTDT) with organotin groups, enabling efficient Stille cross-coupling reactions to construct high-performance semiconducting polymers .
Key applications include:
Preparation Methods
Synthetic Routes and Reaction Conditions
The synthesis of 2,6-Bis(triMethyltin)-dioctyl- typically involves the reaction of dioctyl precursors with trimethyltin chloride in the presence of a suitable base. The reaction is usually carried out in an inert atmosphere to prevent oxidation and hydrolysis of the organotin compound. The reaction conditions often include:
Solvent: Tetrahydrofuran (THF) or toluene
Temperature: Room temperature to reflux
Base: Triethylamine or sodium hydride
Industrial Production Methods
Industrial production of 2,6-Bis(triMethyltin)-dioctyl- follows similar synthetic routes but on a larger scale. The process involves:
Large-scale reactors: Equipped with inert gas purging systems
Continuous monitoring: To ensure the purity and yield of the product
Purification: Techniques such as distillation or recrystallization to obtain high-purity 2,6-Bis(triMethyltin)-dioctyl-
Chemical Reactions Analysis
Types of Reactions
2,6-Bis(triMethyltin)-dioctyl- undergoes various types of chemical reactions, including:
Substitution reactions: Where the trimethyltin groups can be replaced by other nucleophiles
Oxidation reactions: Leading to the formation of organotin oxides
Reduction reactions: Resulting in the formation of lower oxidation state tin compounds
Common Reagents and Conditions
Substitution: Nucleophiles such as halides, amines, or thiols in the presence of a suitable solvent like THF or dichloromethane
Oxidation: Oxidizing agents such as hydrogen peroxide or ozone
Reduction: Reducing agents like lithium aluminum hydride or sodium borohydride
Major Products Formed
Substitution: Formation of new organotin compounds with different functional groups
Oxidation: Formation of organotin oxides
Reduction: Formation of lower oxidation state tin compounds
Scientific Research Applications
Applications in Organic Electronics
2.1 Photovoltaic Devices
One of the primary applications of 2,6-Bis(triMethyltin)-dioctyl- is in organic solar cells (OSCs). The compound has been used to enhance the performance of photovoltaic devices by improving charge transport and reducing recombination losses. Studies have shown that incorporating this compound into polymer blends can lead to increased power conversion efficiencies (PCE) due to better energy level alignment and morphology optimization in the active layer.
Table 1: Performance Metrics of OSCs with 2,6-Bis(triMethyltin)-dioctyl-
Study Reference | PCE (%) | Active Layer Thickness (nm) | Charge Mobility (cm²/Vs) |
---|---|---|---|
8.63 | 300 | Improved with annealing | |
7.50 | 250 | Enhanced by blending |
Case Study:
In a recent study, researchers reported that blending 2,6-Bis(triMethyltin)-dioctyl- with specific donor polymers resulted in optimized morphology and increased charge carrier mobility, leading to a significant boost in device efficiency .
Applications in Material Science
3.1 Synthesis of Conjugated Polymers
The compound serves as a valuable building block in the synthesis of conjugated polymers. Its ability to facilitate cross-coupling reactions has been exploited to create polymers with tailored electronic properties for use in organic light-emitting diodes (OLEDs) and field-effect transistors (FETs).
Table 2: Properties of Polymers Synthesized with 2,6-Bis(triMethyltin)-dioctyl-
Polymer Type | Band Gap (eV) | Solubility | Application Area |
---|---|---|---|
Polythiophene | 1.80 | High | OLEDs |
Dithienosilole | 1.60 | Moderate | FETs |
Case Study:
A study demonstrated that polymers synthesized using 2,6-Bis(triMethyltin)-dioctyl- exhibited enhanced photophysical properties, making them suitable candidates for optoelectronic applications .
Biological Applications
4.1 Anticancer Activity
Recent investigations have explored the biological activity of organotin compounds, including 2,6-Bis(triMethyltin)-dioctyl-, against various cancer cell lines. Preliminary results indicate that these compounds may induce apoptosis in cancer cells through mechanisms involving oxidative stress and mitochondrial dysfunction.
Table 3: Anticancer Activity of Organotin Compounds
Compound | Cell Line | IC50 (µM) | Mechanism |
---|---|---|---|
2,6-Bis(triMethyltin)-dioctyl- | HeLa | <50 | Apoptosis induction |
Other Organotin Derivatives | MCF-7 | <100 | Oxidative stress |
Case Study:
In vitro studies have shown that treatment with organotin compounds leads to significant cytotoxic effects on HeLa cells, suggesting potential for further development as anticancer agents .
Mechanism of Action
The mechanism of action of 2,6-Bis(triMethyltin)-dioctyl- involves its interaction with molecular targets such as enzymes and receptors. The trimethyltin groups can form covalent bonds with nucleophilic sites on proteins and other biomolecules, leading to alterations in their structure and function. This can result in various biological effects, including enzyme inhibition and disruption of cellular processes.
Comparison with Similar Compounds
Structural and Functional Analogues
Table 1: Comparative Analysis of 2,6-Bis(trimethyltin)-dioctyl- Derivatives and Analogues
Key Research Findings
a) Comparison with 2,6-Bis(trimethyltin)pyridine
While both compounds contain a 2,6-bis(trimethyltin) motif, 2,6-Bis(trimethyltin)pyridine replaces the dithiophene core with pyridine. This structural difference drastically alters reactivity:
- The pyridine derivative acts as a supramolecular building block for ligands, leveraging nitrogen’s lone pair for coordination chemistry .
- In contrast, the dithienothiophene analogue prioritizes π-conjugation for charge transport in OPVs .
b) Comparison with Dioctyltin Dilaurate
Dioctyltin dilaurate, a linear organotin compound, is restricted under RoHS due to its endocrine-disrupting toxicity . In contrast, 2,6-Bis(trimethyltin)-dioctyl- derivatives exhibit lower bioaccumulation risks owing to their bulky aromatic cores, making them safer for electronic applications .
c) Comparison with PBDTTT-C-T Polymers
Polymers derived from 2,6-Bis(trimethyltin)-dioctyl- (e.g., PBDTTT-C-T) demonstrate superior vertical phase separation in PSCs compared to PTB7:PC71BM blends. For instance:
- XPS data show a 1:0.48 ED:EA ratio at the surface of PBDTTT-C-T:PC71BM layers (vs. 1:0.88 without additives), indicating polymer enrichment for efficient hole extraction .
Biological Activity
2,6-Bis(triMethyltin)-dioctyl- is an organotin compound that has garnered attention in various fields, particularly in polymer chemistry and biological studies. Organotin compounds are known for their diverse biological activities, including neurotoxicity and potential endocrine disruption. This article provides a detailed overview of the biological activity of 2,6-Bis(triMethyltin)-dioctyl-, supported by data tables, case studies, and research findings.
Chemical Structure
The structure of 2,6-Bis(triMethyltin)-dioctyl- can be represented as follows:
This compound features two tri-methyltin groups attached to a dioctyl backbone, which influences its solubility and biological interactions.
Biological Activity Overview
Organotin compounds like 2,6-Bis(triMethyltin)-dioctyl- exhibit a range of biological activities, primarily due to their ability to interact with cellular membranes and proteins. The following sections detail specific activities observed in various studies.
Neurotoxicity
Research indicates that organotin compounds can induce neurotoxic effects. For instance, a study comparing different organotins found that dibutyltin (DBT) exhibited significant neurotoxicity in vitro, suggesting similar potential for other organotins like 2,6-Bis(triMethyltin)-dioctyl- due to structural similarities .
Case Study: Neurotoxic Effects
A study evaluated the neurotoxic effects of various organotin compounds on human neuroblastoma cell lines. The findings indicated that exposure to these compounds led to increased production of reactive oxygen species (ROS) and subsequent cell death. The specific mechanisms included apoptosis and necrosis pathways being activated at varying concentrations .
Endocrine Disruption
Organotins are known endocrine disruptors. Research has shown that certain organotin compounds can interfere with hormonal signaling pathways. A study on the effects of tributyltin (TBT), a related compound, revealed alterations in reproductive development in gastropods, suggesting that 2,6-Bis(triMethyltin)-dioctyl- may exhibit similar disruptive properties .
Data Tables
The biological activity of 2,6-Bis(triMethyltin)-dioctyl- can be attributed to several mechanisms:
- Membrane Interaction : Organotin compounds can integrate into cell membranes, altering fluidity and function.
- Oxidative Stress : Increased ROS production leads to cellular damage and apoptosis.
- Hormonal Interference : By mimicking or blocking hormone receptors, organotins can disrupt normal endocrine functions.
Research Findings
A comprehensive review of literature reveals a consistent pattern of toxicity associated with organotin compounds:
- Toxicity Studies : In vivo studies have demonstrated that exposure to organotins results in significant neurotoxic effects at low concentrations.
- Environmental Impact : Organotins persist in the environment due to their stability and lipophilicity, leading to bioaccumulation and long-term ecological consequences.
Q & A
Basic Research Questions
Q. What are the recommended synthetic routes for 2,6-Bis(trimethyltin)-dioctyl- derivatives, and how can purity be optimized?
- Methodology : The synthesis typically involves Stille coupling reactions, where trimethyltin groups act as nucleophiles. Key steps include:
- Using palladium catalysts (e.g., Pd(PPh₃)₄) under inert atmospheres to prevent tin oxidation .
- Purification via column chromatography with non-polar solvents (hexane/ethyl acetate) to isolate the product.
- Purity validation through elemental analysis (>98% purity) and NMR to confirm absence of residual tin byproducts .
- Critical Parameters : Moisture-sensitive intermediates require anhydrous conditions. Tin-based reagents may require low temperatures (−20°C) to stabilize reactive intermediates.
Q. How should researchers characterize the structural and electronic properties of 2,6-Bis(trimethyltin)-dioctyl- derivatives?
- Analytical Techniques :
- ¹H/¹³C NMR : Identify proton environments (e.g., aromatic vs. aliphatic regions) and confirm substitution patterns. Tin satellites in NMR indicate coupling between Sn and adjacent protons .
- X-ray Crystallography : Resolve crystal packing and bond angles, critical for understanding steric effects in optoelectronic applications .
- Elemental Analysis : Validate stoichiometry (e.g., C₁₄H₂₀S₃Sn₂) and rule out contaminants like unreacted tin precursors .
- Data Table :
Technique | Expected Outcome (Example) | Reference |
---|---|---|
¹H NMR (CDCl₃) | δ 0.4–0.6 ppm (Sn–CH₃) | |
X-ray | Dihedral angle ~160° between thiophene rings |
Q. What are the primary applications of 2,6-Bis(trimethyltin)-dioctyl- derivatives in materials science?
- Optoelectronic Devices : Used as electron-deficient monomers in organic photovoltaics (OPV) and organic thin-film transistors (OTFTs) due to their high electron mobility and air stability .
- Experimental Design :
- Blend with electron-rich polymers (e.g., P3HT) in bulk heterojunction solar cells.
- Optimize annealing temperatures (e.g., 150°C) to enhance crystallinity and charge transport .
Advanced Research Questions
Q. How do structural modifications (e.g., alkyl chain length) impact the performance of 2,6-Bis(trimethyltin)-dioctyl- derivatives in OPVs?
- Mechanistic Insight : Longer alkyl chains (e.g., dioctyl vs. dihexyl) improve solubility but may reduce crystallinity, leading to trade-offs between film morphology and charge mobility.
- Data Contradiction Analysis :
- Some studies report increased power conversion efficiency (PCE) with longer chains due to reduced aggregation , while others note lower PCE from diminished π-π stacking.
- Resolution: Use grazing-incidence X-ray scattering (GIWAXS) to correlate chain length with domain size and phase separation .
Q. What are the key challenges in stabilizing 2,6-Bis(trimethyltin)-dioctyl- derivatives during storage and device fabrication?
- Degradation Pathways :
- Hydrolysis of Sn–C bonds in humid environments.
- Oxidation under ambient light/oxygen, forming tin oxides.
- Mitigation Strategies :
- Store compounds in argon-filled gloveboxes with molecular sieves.
- Incorporate UV stabilizers (e.g., hindered amine light stabilizers) in device encapsulation .
Q. How can computational modeling guide the design of 2,6-Bis(trimethyltin)-dioctyl- derivatives for specific electronic properties?
- Methods :
- DFT calculations (e.g., B3LYP/6-31G*) to predict HOMO/LUMO levels and bandgap tuning.
- Molecular dynamics (MD) simulations to assess packing efficiency in thin films.
- Case Study : Simulations predicting a 0.3 eV reduction in LUMO with electron-withdrawing substituents align with experimental OPV performance .
Q. What regulatory considerations apply to the use of tin-containing compounds like 2,6-Bis(trimethyltin)-dioctyl- derivatives?
Properties
Molecular Formula |
C56H90S4Sn2 |
---|---|
Molecular Weight |
1129.0 g/mol |
IUPAC Name |
[4,8-bis(4,5-dioctylthiophen-2-yl)-2-trimethylstannylthieno[2,3-f][1]benzothiol-6-yl]-trimethylstannane |
InChI |
InChI=1S/C50H72S4.6CH3.2Sn/c1-5-9-13-17-21-25-29-39-37-45(53-43(39)31-27-23-19-15-11-7-3)47-41-33-35-52-50(41)48(42-34-36-51-49(42)47)46-38-40(30-26-22-18-14-10-6-2)44(54-46)32-28-24-20-16-12-8-4;;;;;;;;/h33-34,37-38H,5-32H2,1-4H3;6*1H3;; |
InChI Key |
LYAUAXDJHZCGBI-UHFFFAOYSA-N |
Canonical SMILES |
CCCCCCCCC1=C(SC(=C1)C2=C3C=C(SC3=C(C4=C2SC(=C4)[Sn](C)(C)C)C5=CC(=C(S5)CCCCCCCC)CCCCCCCC)[Sn](C)(C)C)CCCCCCCC |
Origin of Product |
United States |
Disclaimer and Information on In-Vitro Research Products
Please be aware that all articles and product information presented on BenchChem are intended solely for informational purposes. The products available for purchase on BenchChem are specifically designed for in-vitro studies, which are conducted outside of living organisms. In-vitro studies, derived from the Latin term "in glass," involve experiments performed in controlled laboratory settings using cells or tissues. It is important to note that these products are not categorized as medicines or drugs, and they have not received approval from the FDA for the prevention, treatment, or cure of any medical condition, ailment, or disease. We must emphasize that any form of bodily introduction of these products into humans or animals is strictly prohibited by law. It is essential to adhere to these guidelines to ensure compliance with legal and ethical standards in research and experimentation.