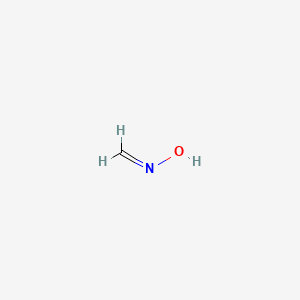
Formaldoxime
Overview
Description
Formaldoxime is an organic compound with the chemical formula H₂C=N−OH. It is the oxime derivative of formaldehyde and appears as a colorless liquid. The pure compound tends to polymerize into a cyclic trimer, but it remains stable in aqueous solutions and as this compound hydrochloride ([H₂C=N(−H)(−OH)]⁺Cl⁻) . This compound is primarily used as a reagent in organic synthesis, particularly for converting aryl diazonium salts to aryl aldehydes .
Preparation Methods
Formaldoxime is synthesized by combining hydroxylamine and formaldehyde . The reaction typically involves the following steps:
Hydroxylamine Hydrochloride Preparation: Hydroxylamine hydrochloride is prepared by reacting sodium nitrite with ammonium chloride in the presence of hydrochloric acid.
Reaction with Formaldehyde: Hydroxylamine hydrochloride is then reacted with formaldehyde in an aqueous solution to produce this compound.
Industrial production methods for this compound are similar, involving the controlled reaction of hydroxylamine and formaldehyde under specific conditions to ensure high yield and purity .
Chemical Reactions Analysis
Formaldoxime undergoes several types of chemical reactions, including:
Oxidation: this compound can be oxidized to formic acid and nitrogen gas.
Reduction: It can be reduced to formaldehyde and hydroxylamine.
Substitution: This compound reacts with aryl diazonium salts to form aryl aldehydes.
Common reagents and conditions used in these reactions include oxidizing agents like potassium permanganate for oxidation, reducing agents like sodium borohydride for reduction, and aryl diazonium salts for substitution reactions. The major products formed from these reactions are formic acid, formaldehyde, and aryl aldehydes .
Scientific Research Applications
Formaldoxime has several scientific research applications:
Mechanism of Action
The mechanism of action of formaldoxime involves its reactivity with various chemical species. For example, in the conversion of aryl diazonium salts to aryl aldehydes, this compound acts as a nucleophile, attacking the diazonium ion to form the corresponding aldehyde . The molecular targets and pathways involved in these reactions depend on the specific chemical context in which this compound is used.
Comparison with Similar Compounds
Formaldoxime is similar to other oximes, such as acetaldoxime and benzaldoxime, which are derived from acetaldehyde and benzaldehyde, respectively . this compound is unique due to its simple structure and high reactivity. It is particularly useful in organic synthesis for converting aryl diazonium salts to aryl aldehydes, a reaction not commonly performed by other oximes .
Similar Compounds
Acetaldoxime: Derived from acetaldehyde.
Benzaldoxime: Derived from benzaldehyde.
Phosgene Oxime: A toxic compound used in chemical warfare.
Properties
IUPAC Name |
N-methylidenehydroxylamine | |
---|---|---|
Source | PubChem | |
URL | https://pubchem.ncbi.nlm.nih.gov | |
Description | Data deposited in or computed by PubChem | |
InChI |
InChI=1S/CH3NO/c1-2-3/h3H,1H2 | |
Source | PubChem | |
URL | https://pubchem.ncbi.nlm.nih.gov | |
Description | Data deposited in or computed by PubChem | |
InChI Key |
SQDFHQJTAWCFIB-UHFFFAOYSA-N | |
Source | PubChem | |
URL | https://pubchem.ncbi.nlm.nih.gov | |
Description | Data deposited in or computed by PubChem | |
Canonical SMILES |
C=NO | |
Source | PubChem | |
URL | https://pubchem.ncbi.nlm.nih.gov | |
Description | Data deposited in or computed by PubChem | |
Molecular Formula |
CH3NO | |
Source | PubChem | |
URL | https://pubchem.ncbi.nlm.nih.gov | |
Description | Data deposited in or computed by PubChem | |
DSSTOX Substance ID |
DTXSID9058785 | |
Record name | Nitrone | |
Source | EPA DSSTox | |
URL | https://comptox.epa.gov/dashboard/DTXSID9058785 | |
Description | DSSTox provides a high quality public chemistry resource for supporting improved predictive toxicology. | |
Molecular Weight |
45.041 g/mol | |
Source | PubChem | |
URL | https://pubchem.ncbi.nlm.nih.gov | |
Description | Data deposited in or computed by PubChem | |
CAS No. |
75-17-2 | |
Record name | Nitrone | |
Source | CAS Common Chemistry | |
URL | https://commonchemistry.cas.org/detail?cas_rn=75-17-2 | |
Description | CAS Common Chemistry is an open community resource for accessing chemical information. Nearly 500,000 chemical substances from CAS REGISTRY cover areas of community interest, including common and frequently regulated chemicals, and those relevant to high school and undergraduate chemistry classes. This chemical information, curated by our expert scientists, is provided in alignment with our mission as a division of the American Chemical Society. | |
Explanation | The data from CAS Common Chemistry is provided under a CC-BY-NC 4.0 license, unless otherwise stated. | |
Record name | Formaldoxime | |
Source | ChemIDplus | |
URL | https://pubchem.ncbi.nlm.nih.gov/substance/?source=chemidplus&sourceid=0000075172 | |
Description | ChemIDplus is a free, web search system that provides access to the structure and nomenclature authority files used for the identification of chemical substances cited in National Library of Medicine (NLM) databases, including the TOXNET system. | |
Record name | Formaldehyde, oxime | |
Source | EPA Chemicals under the TSCA | |
URL | https://www.epa.gov/chemicals-under-tsca | |
Description | EPA Chemicals under the Toxic Substances Control Act (TSCA) collection contains information on chemicals and their regulations under TSCA, including non-confidential content from the TSCA Chemical Substance Inventory and Chemical Data Reporting. | |
Record name | Nitrone | |
Source | EPA DSSTox | |
URL | https://comptox.epa.gov/dashboard/DTXSID9058785 | |
Description | DSSTox provides a high quality public chemistry resource for supporting improved predictive toxicology. | |
Record name | Formaldehyde oxime | |
Source | European Chemicals Agency (ECHA) | |
URL | https://echa.europa.eu/substance-information/-/substanceinfo/100.000.769 | |
Description | The European Chemicals Agency (ECHA) is an agency of the European Union which is the driving force among regulatory authorities in implementing the EU's groundbreaking chemicals legislation for the benefit of human health and the environment as well as for innovation and competitiveness. | |
Explanation | Use of the information, documents and data from the ECHA website is subject to the terms and conditions of this Legal Notice, and subject to other binding limitations provided for under applicable law, the information, documents and data made available on the ECHA website may be reproduced, distributed and/or used, totally or in part, for non-commercial purposes provided that ECHA is acknowledged as the source: "Source: European Chemicals Agency, http://echa.europa.eu/". Such acknowledgement must be included in each copy of the material. ECHA permits and encourages organisations and individuals to create links to the ECHA website under the following cumulative conditions: Links can only be made to webpages that provide a link to the Legal Notice page. | |
Record name | FORMALDOXIME | |
Source | FDA Global Substance Registration System (GSRS) | |
URL | https://gsrs.ncats.nih.gov/ginas/app/beta/substances/420JFM0Z1Q | |
Description | The FDA Global Substance Registration System (GSRS) enables the efficient and accurate exchange of information on what substances are in regulated products. Instead of relying on names, which vary across regulatory domains, countries, and regions, the GSRS knowledge base makes it possible for substances to be defined by standardized, scientific descriptions. | |
Explanation | Unless otherwise noted, the contents of the FDA website (www.fda.gov), both text and graphics, are not copyrighted. They are in the public domain and may be republished, reprinted and otherwise used freely by anyone without the need to obtain permission from FDA. Credit to the U.S. Food and Drug Administration as the source is appreciated but not required. | |
Retrosynthesis Analysis
AI-Powered Synthesis Planning: Our tool employs the Template_relevance Pistachio, Template_relevance Bkms_metabolic, Template_relevance Pistachio_ringbreaker, Template_relevance Reaxys, Template_relevance Reaxys_biocatalysis model, leveraging a vast database of chemical reactions to predict feasible synthetic routes.
One-Step Synthesis Focus: Specifically designed for one-step synthesis, it provides concise and direct routes for your target compounds, streamlining the synthesis process.
Accurate Predictions: Utilizing the extensive PISTACHIO, BKMS_METABOLIC, PISTACHIO_RINGBREAKER, REAXYS, REAXYS_BIOCATALYSIS database, our tool offers high-accuracy predictions, reflecting the latest in chemical research and data.
Strategy Settings
Precursor scoring | Relevance Heuristic |
---|---|
Min. plausibility | 0.01 |
Model | Template_relevance |
Template Set | Pistachio/Bkms_metabolic/Pistachio_ringbreaker/Reaxys/Reaxys_biocatalysis |
Top-N result to add to graph | 6 |
Feasible Synthetic Routes
Q1: What is the molecular formula and weight of formaldoxime?
A1: this compound has a molecular formula of CH2NOH and a molecular weight of 45.04 g/mol. []
Q2: What are some key spectroscopic features of this compound?
A2: this compound exhibits characteristic infrared absorption bands corresponding to its various vibrational modes. For instance, the ν4, ν5, ν6, and ν8 fundamental bands have been observed and analyzed in detail, providing insights into its molecular structure and dynamics. [] Additionally, the third harmonic of the O-H stretching vibration has been identified at λ9572 (10,444.1 cm-1). [, ] High-resolution rotational spectroscopy studies have also provided precise information about its rotational constants, 14N quadrupole coupling constants, and spin-rotation coupling constants. []
Q3: Does this compound exhibit isomerism?
A3: Yes, this compound exists as two isomers: cis and trans. The trans isomer is generally more stable. [, , , ] The energy difference between the two isomers, as well as the energy barriers for their interconversion, have been investigated using computational methods. [, ]
Q4: What is the structure of this compound?
A4: this compound is a planar molecule. Studies utilizing isotopic substitution and microwave spectroscopy determined the following bond lengths (in Angstroms): CHcis = 1.085, CHtrans = 1.086, C=N = 1.276, NO = 1.408, OH = 0.956. Bond angles were also determined: HcisCN 121°46′, HtransCN 115°33′, CNO 110°12′, NOH 102°41′. An interesting feature is the difference between the cis- and trans-HCN bond angles. []
Q5: How does this compound react with nitrous acid?
A5: this compound reacts with nitrous acid (HNO2) in an acidified solution. The kinetics of this reaction have been studied, revealing a rate equation of: -dc(HNO2)/dt=kc(HNO2)c1.32(FO)c0.96(ClO-4), where FO represents this compound. The reaction rate is influenced by the concentrations of this compound, perchlorate ions, and temperature but not significantly affected by H+ concentration. []
Q6: Can this compound be used to detect manganese?
A6: Yes, this compound forms a colored complex with manganese ions, forming the basis for a spectrophotometric method for manganese detection. This method is frequently used for determining manganese concentrations in various matrices, including water, textiles, soil, and silicate rocks. [, , , , , , ]
Q7: How can iron interference in the this compound method for manganese determination be addressed?
A7: Iron can interfere with manganese determination using the this compound method because it also forms a complex with the reagent. Several approaches have been explored to mitigate this interference, including the use of EDTA to selectively decompose the iron-formaldoxime complex [, ], the application of derivative spectrophotometry to isolate the spectral signal of manganese [], and the separation of iron from the sample matrix using techniques such as extraction with methylene chloride or passage through a cellulose phosphate column. [, ]
Q8: What is the role of this compound in the pyrolysis of nitrosomethane?
A8: this compound is a proposed intermediate in the pyrolysis of nitrosomethane (CH3NO). It is suggested that methyl radicals generated during the process can react with nitric oxide to form a CH3NO complex, which can then isomerize to this compound. this compound itself decomposes to hydrogen cyanide and water. []
Q9: How is this compound involved in the study of methylamidogen radical fluorescence?
A9: this compound and its methylated derivatives have been used as precursors for generating methylamidogen radicals (R1R2CN, where R1, R2 = H or CH3) in photodissociation experiments. Upon irradiation at 193 nm, this compound decomposes, producing the H2CN radical alongside other products. These studies provided insights into the photochemistry of these species and yielded valuable information about the radicals' excited states and dissociation dynamics. []
Q10: How has computational chemistry been used to study this compound?
A10: Computational methods, including density functional theory (DFT) and coupled-cluster theory, have been employed to calculate various molecular properties of this compound, including optimized geometries, vibrational frequencies, anharmonic force fields, and potential energy surfaces. These calculations have helped in understanding the conformational preferences, spectroscopic features, and reaction pathways of this compound and its isomers. [, , , , ]
Q11: Have there been computational studies on the interaction of this compound with metal ions?
A11: Yes, computational studies have explored the interaction of this compound with metal ions like Cu+. These studies have investigated the relative stability of different complexation modes, providing insights into the coordination chemistry of this compound. []
Q12: How can this compound be synthesized?
A13: While not explicitly detailed in the provided papers, this compound can be synthesized by reacting formaldehyde with hydroxylamine. []
Q13: Can you describe a specific synthetic application of this compound?
A14: this compound, in its O-benzylated form (this compound-O-benzylether), can be used to synthesize 1-benzyloxyaminomethyl phosphonic and phosphinic acids. This reaction involves the use of phosphorus trichloride and dichlorophosphines, highlighting the versatility of this compound derivatives in organic synthesis. []
Disclaimer and Information on In-Vitro Research Products
Please be aware that all articles and product information presented on BenchChem are intended solely for informational purposes. The products available for purchase on BenchChem are specifically designed for in-vitro studies, which are conducted outside of living organisms. In-vitro studies, derived from the Latin term "in glass," involve experiments performed in controlled laboratory settings using cells or tissues. It is important to note that these products are not categorized as medicines or drugs, and they have not received approval from the FDA for the prevention, treatment, or cure of any medical condition, ailment, or disease. We must emphasize that any form of bodily introduction of these products into humans or animals is strictly prohibited by law. It is essential to adhere to these guidelines to ensure compliance with legal and ethical standards in research and experimentation.