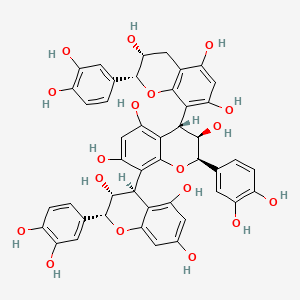
Procyanidin C1
Overview
Description
Procyanidin C1 is a type of B-type proanthocyanidin, specifically an epicatechin trimer. It is naturally found in various plants, including grape (Vitis vinifera), unripe apples, and cinnamon . This compound is known for its significant health benefits, including antioxidant, anti-inflammatory, and anti-cancer properties .
Mechanism of Action
Target of Action
Procyanidin C1 (PC1) is a trimeric procyanidin known for its numerous health benefits . It primarily targets adipocytes, enhancing insulin sensitivity . It also interacts with biomembranes, modulating their structure and dynamics . Furthermore, it targets senescent cells, inducing their apoptosis .
Mode of Action
PC1 interacts with its targets in several ways. In adipocytes, it activates the AKT-eNOS pathway, up-regulating glucose uptake and enhancing insulin sensitivity . In biomembranes, PC1 tends to locate at the membrane interphase, interacting with lipids and proteins, and modulating the structure and dynamics of the membrane . In senescent cells, PC1 induces apoptosis selectively .
Biochemical Pathways
PC1 affects several biochemical pathways. It activates the AKT-eNOS pathway in adipocytes . In senescent cells, it doesn’t affect the DNA damage response but activates the p53-p38 MAPK apoptosis signaling pathway . It also increases the accumulation of reactive oxygen species (ROS) in senescent cells, induces a decrease in mitochondrial membrane potential, and promotes the release of cytochrome c from mitochondria, leading to cell apoptosis .
Pharmacokinetics
Due to its relatively high lipid–water partition coefficient, it is believed to interact with the biomembrane, which could influence its bioavailability .
Result of Action
The action of PC1 results in several molecular and cellular effects. It enhances insulin sensitivity in adipocytes , modulates the structure and dynamics of biomembranes , and induces apoptosis in senescent cells . These effects contribute to its potential health benefits, including its antioxidant, anticancer, anti-inflammatory, immunosuppressive, and antiallergy properties .
Action Environment
The action of PC1 can be influenced by environmental factors. For instance, its antioxidant activity functions as a protective mechanism against oxidative stress caused by reactive oxygen species (ROS) in the environment
Biochemical Analysis
Biochemical Properties
Procyanidin C1 plays a crucial role in biochemical reactions, primarily due to its interaction with various enzymes, proteins, and other biomolecules. It has been shown to interact with membrane lipids, modulating the structure and dynamics of biomembranes . Additionally, this compound can enhance the production of reactive oxygen species (ROS) and disturb mitochondrial membrane potential, leading to the upregulation of pro-apoptotic factors such as Puma and Noxa . These interactions highlight the compound’s potential in influencing cellular processes and signaling pathways.
Cellular Effects
This compound exhibits a range of effects on different cell types and cellular processes. It has been found to selectively kill senescent cells by enhancing ROS production and disturbing mitochondrial membrane potential . This compound also activates the Nrf2/HO-1 signaling pathway, which helps in preventing oxidative stress . Furthermore, this compound has been shown to inhibit adipogenesis, melanogenesis, and oxidative stress, while enhancing lipid metabolism and macrophage activity . These cellular effects underscore the compound’s potential in modulating cell function and metabolism.
Molecular Mechanism
At the molecular level, this compound exerts its effects through various mechanisms. It interacts with membrane lipids, modulating their structure and dynamics . The compound also enhances ROS production and disturbs mitochondrial membrane potential, leading to the upregulation of pro-apoptotic factors such as Puma and Noxa . Additionally, this compound activates the Nrf2/HO-1 signaling pathway, which plays a crucial role in preventing oxidative stress . These molecular interactions and mechanisms contribute to the compound’s overall bioactivity.
Temporal Effects in Laboratory Settings
In laboratory settings, the effects of this compound have been observed to change over time. The compound has been shown to block the expression of the senescence-associated secretory phenotype (SASP) at low concentrations, while selectively killing senescent cells at higher concentrations . These temporal effects highlight the compound’s potential in mediating anti-aging effects and improving cellular function over time.
Dosage Effects in Animal Models
The effects of this compound vary with different dosages in animal models. At low concentrations, the compound blocks SASP expression, while at higher concentrations, it selectively kills senescent cells These dosage-dependent effects suggest that this compound can be used to modulate cellular senescence and improve healthspan in animal models
Metabolic Pathways
This compound is involved in various metabolic pathways, interacting with enzymes and cofactors. It has been shown to enhance ROS production and disturb mitochondrial membrane potential, leading to the upregulation of pro-apoptotic factors . Additionally, the compound activates the Nrf2/HO-1 signaling pathway, which plays a crucial role in preventing oxidative stress . These interactions highlight the compound’s involvement in metabolic processes and its potential impact on metabolic flux and metabolite levels.
Transport and Distribution
This compound is transported and distributed within cells and tissues through interactions with membrane lipids . The compound has a high lipid-water partition coefficient, allowing it to modulate the structure and dynamics of biomembranes . This property facilitates its transport and distribution within cells, influencing its localization and accumulation.
Subcellular Localization
This compound tends to localize at the membrane interphase, with part of the molecule exposed to the water solvent and part reaching the first carbons of the hydrocarbon chains . This localization allows the compound to interact with membrane lipids and proteins, modulating their structure and dynamics . These subcellular interactions contribute to the compound’s bioactivity and potential therapeutic applications.
Preparation Methods
Synthetic Routes and Reaction Conditions: The stereoselective synthesis of procyanidin C1 involves the condensation of epicatechin units. One common method is the TMSOTf-catalyzed condensation reaction, which yields benzylated proanthocyanidin trimers. The final deprotection step gives natural procyanidin trimers in good yields .
Industrial Production Methods: this compound can be isolated from grape seed extract using reversed-phase column chromatography . This method allows for the separation and purification of this compound from other components in the extract.
Chemical Reactions Analysis
Types of Reactions: Procyanidin C1 undergoes various chemical reactions, including oxidation, reduction, and substitution. These reactions are essential for modifying the compound’s structure and enhancing its bioactivity.
Common Reagents and Conditions:
Oxidation: Common oxidizing agents include hydrogen peroxide and potassium permanganate.
Reduction: Reducing agents such as sodium borohydride are used.
Substitution: Various nucleophiles can be used for substitution reactions, depending on the desired modification.
Major Products: The major products formed from these reactions include modified procyanidin derivatives with enhanced biological activities.
Scientific Research Applications
Procyanidin C1 has a wide range of scientific research applications:
Chemistry: Used as a model compound for studying the properties and reactions of proanthocyanidins.
Biology: Investigated for its role in modulating cellular processes and signaling pathways.
Industry: Utilized in the food and cosmetic industries for its antioxidant properties.
Comparison with Similar Compounds
Procyanidin B1: A dimer of epicatechin with similar antioxidant properties.
Procyanidin B2: Another dimer of epicatechin, known for its anti-inflammatory and anti-cancer activities.
Procyanidin C2: A trimer of catechin with comparable health benefits.
Uniqueness: Procyanidin C1 is unique due to its specific trimeric structure, which enhances its bioactivity and allows for more potent health benefits compared to its dimeric counterparts .
Properties
IUPAC Name |
(2R,3R,4S)-2-(3,4-dihydroxyphenyl)-4-[(2R,3R)-2-(3,4-dihydroxyphenyl)-3,5,7-trihydroxy-3,4-dihydro-2H-chromen-8-yl]-8-[(2R,3R,4R)-2-(3,4-dihydroxyphenyl)-3,5,7-trihydroxy-3,4-dihydro-2H-chromen-4-yl]-3,4-dihydro-2H-chromene-3,5,7-triol | |
---|---|---|
Source | PubChem | |
URL | https://pubchem.ncbi.nlm.nih.gov | |
Description | Data deposited in or computed by PubChem | |
InChI |
InChI=1S/C45H38O18/c46-18-10-27(54)33-32(11-18)61-42(16-2-5-21(48)25(52)8-16)39(59)37(33)35-29(56)14-30(57)36-38(40(60)43(63-45(35)36)17-3-6-22(49)26(53)9-17)34-28(55)13-23(50)19-12-31(58)41(62-44(19)34)15-1-4-20(47)24(51)7-15/h1-11,13-14,31,37-43,46-60H,12H2/t31-,37-,38+,39-,40-,41-,42-,43-/m1/s1 | |
Source | PubChem | |
URL | https://pubchem.ncbi.nlm.nih.gov | |
Description | Data deposited in or computed by PubChem | |
InChI Key |
MOJZMWJRUKIQGL-XILRTYJMSA-N | |
Source | PubChem | |
URL | https://pubchem.ncbi.nlm.nih.gov | |
Description | Data deposited in or computed by PubChem | |
Canonical SMILES |
C1C(C(OC2=C1C(=CC(=C2C3C(C(OC4=C(C(=CC(=C34)O)O)C5C(C(OC6=CC(=CC(=C56)O)O)C7=CC(=C(C=C7)O)O)O)C8=CC(=C(C=C8)O)O)O)O)O)C9=CC(=C(C=C9)O)O)O | |
Source | PubChem | |
URL | https://pubchem.ncbi.nlm.nih.gov | |
Description | Data deposited in or computed by PubChem | |
Isomeric SMILES |
C1[C@H]([C@H](OC2=C1C(=CC(=C2[C@@H]3[C@H]([C@H](OC4=C(C(=CC(=C34)O)O)[C@@H]5[C@H]([C@H](OC6=CC(=CC(=C56)O)O)C7=CC(=C(C=C7)O)O)O)C8=CC(=C(C=C8)O)O)O)O)O)C9=CC(=C(C=C9)O)O)O | |
Source | PubChem | |
URL | https://pubchem.ncbi.nlm.nih.gov | |
Description | Data deposited in or computed by PubChem | |
Molecular Formula |
C45H38O18 | |
Source | PubChem | |
URL | https://pubchem.ncbi.nlm.nih.gov | |
Description | Data deposited in or computed by PubChem | |
DSSTOX Substance ID |
DTXSID10190562 | |
Record name | Procyanidin C1 | |
Source | EPA DSSTox | |
URL | https://comptox.epa.gov/dashboard/DTXSID10190562 | |
Description | DSSTox provides a high quality public chemistry resource for supporting improved predictive toxicology. | |
Molecular Weight |
866.8 g/mol | |
Source | PubChem | |
URL | https://pubchem.ncbi.nlm.nih.gov | |
Description | Data deposited in or computed by PubChem | |
Physical Description |
Solid | |
Record name | Procyanidin C1 | |
Source | Human Metabolome Database (HMDB) | |
URL | http://www.hmdb.ca/metabolites/HMDB0038370 | |
Description | The Human Metabolome Database (HMDB) is a freely available electronic database containing detailed information about small molecule metabolites found in the human body. | |
Explanation | HMDB is offered to the public as a freely available resource. Use and re-distribution of the data, in whole or in part, for commercial purposes requires explicit permission of the authors and explicit acknowledgment of the source material (HMDB) and the original publication (see the HMDB citing page). We ask that users who download significant portions of the database cite the HMDB paper in any resulting publications. | |
CAS No. |
37064-30-5 | |
Record name | Procyanidin C1 | |
Source | CAS Common Chemistry | |
URL | https://commonchemistry.cas.org/detail?cas_rn=37064-30-5 | |
Description | CAS Common Chemistry is an open community resource for accessing chemical information. Nearly 500,000 chemical substances from CAS REGISTRY cover areas of community interest, including common and frequently regulated chemicals, and those relevant to high school and undergraduate chemistry classes. This chemical information, curated by our expert scientists, is provided in alignment with our mission as a division of the American Chemical Society. | |
Explanation | The data from CAS Common Chemistry is provided under a CC-BY-NC 4.0 license, unless otherwise stated. | |
Record name | Procyanidin C1 | |
Source | ChemIDplus | |
URL | https://pubchem.ncbi.nlm.nih.gov/substance/?source=chemidplus&sourceid=0037064305 | |
Description | ChemIDplus is a free, web search system that provides access to the structure and nomenclature authority files used for the identification of chemical substances cited in National Library of Medicine (NLM) databases, including the TOXNET system. | |
Record name | Procyanidin C1 | |
Source | EPA DSSTox | |
URL | https://comptox.epa.gov/dashboard/DTXSID10190562 | |
Description | DSSTox provides a high quality public chemistry resource for supporting improved predictive toxicology. | |
Record name | PROCYANIDIN C1 | |
Source | FDA Global Substance Registration System (GSRS) | |
URL | https://gsrs.ncats.nih.gov/ginas/app/beta/substances/33516LCW4F | |
Description | The FDA Global Substance Registration System (GSRS) enables the efficient and accurate exchange of information on what substances are in regulated products. Instead of relying on names, which vary across regulatory domains, countries, and regions, the GSRS knowledge base makes it possible for substances to be defined by standardized, scientific descriptions. | |
Explanation | Unless otherwise noted, the contents of the FDA website (www.fda.gov), both text and graphics, are not copyrighted. They are in the public domain and may be republished, reprinted and otherwise used freely by anyone without the need to obtain permission from FDA. Credit to the U.S. Food and Drug Administration as the source is appreciated but not required. | |
Record name | Procyanidin C1 | |
Source | Human Metabolome Database (HMDB) | |
URL | http://www.hmdb.ca/metabolites/HMDB0038370 | |
Description | The Human Metabolome Database (HMDB) is a freely available electronic database containing detailed information about small molecule metabolites found in the human body. | |
Explanation | HMDB is offered to the public as a freely available resource. Use and re-distribution of the data, in whole or in part, for commercial purposes requires explicit permission of the authors and explicit acknowledgment of the source material (HMDB) and the original publication (see the HMDB citing page). We ask that users who download significant portions of the database cite the HMDB paper in any resulting publications. | |
Melting Point |
140.0 - 142.0 °C | |
Record name | Procyanidin C1 | |
Source | Human Metabolome Database (HMDB) | |
URL | http://www.hmdb.ca/metabolites/HMDB0038370 | |
Description | The Human Metabolome Database (HMDB) is a freely available electronic database containing detailed information about small molecule metabolites found in the human body. | |
Explanation | HMDB is offered to the public as a freely available resource. Use and re-distribution of the data, in whole or in part, for commercial purposes requires explicit permission of the authors and explicit acknowledgment of the source material (HMDB) and the original publication (see the HMDB citing page). We ask that users who download significant portions of the database cite the HMDB paper in any resulting publications. | |
Q1: How does Procyanidin C1 interact with cells to exert its biological effects?
A1: this compound has been shown to bind to specific cell surface receptors. For instance, it binds to the 67-kDa laminin receptor (67LR) with a Kd value of 2.8 μM. [] This interaction triggers downstream signaling cascades involving protein kinase A (PKA) and protein phosphatase 2A (PP2A), leading to various cellular responses. []
Q2: What are the downstream effects of this compound binding to the 67LR receptor?
A2: Upon binding to 67LR, this compound initiates a signaling cascade that leads to the dephosphorylation of C-kinase potentiated protein phosphatase-1 inhibitor protein of 17 kDa (CPI17) and myosin regulatory light chain (MRLC) proteins. [] This dephosphorylation subsequently induces actin cytoskeleton remodeling and influences cell growth and behavior. []
Q3: Does this compound interact with any other cellular targets besides 67LR?
A3: While 67LR is a confirmed target, research suggests that this compound may interact with other cellular components. For example, it exhibits antioxidant activity by scavenging free radicals and modulating the expression of antioxidant enzymes like glutathione peroxidase (GSH-Px), catalase (CAT), and superoxide dismutase (SOD). [, ] Further research is needed to fully elucidate its complete interactome.
Q4: What is the molecular formula and weight of this compound?
A4: this compound possesses the molecular formula C45H38O18 and a molecular weight of 866.72 g/mol.
Q5: Are there any spectroscopic data available to characterize this compound?
A5: Yes, this compound has been extensively characterized using spectroscopic techniques. Researchers commonly employ techniques like 1H NMR, 13C NMR, and mass spectrometry (MS) to confirm its structure and purity. [, , , , ] Low-temperature NMR has proven particularly useful in elucidating the stereochemistry of its interflavanoid bonds. [, ]
Q6: Does this compound interact with food matrices and impact its bioaccessibility?
A6: this compound can interact with components in food matrices, which might influence its bioavailability. For instance, it exhibits a strong binding affinity for proteins like bovine serum albumin (BSA). [] Such interactions can affect its absorption and overall efficacy.
Q7: Does this compound exhibit any catalytic activities?
A7: While this compound is not typically recognized for direct catalytic properties, its interaction with enzymes can influence their activities. Notably, it inhibits the hydrolysis of starch by pancreatin, with a more pronounced effect on potato starch compared to corn starch. [] This inhibitory effect is suggested to be influenced by both the intact this compound molecule and its degradation products, with the specific mechanism depending on the type of starch being hydrolyzed. []
Q8: Have computational methods been used to study this compound?
A8: Yes, computational chemistry and modeling tools are being increasingly employed in this compound research. Molecular docking simulations have been used to investigate its binding affinity to various targets, including enzymes and receptors. [, , ] These simulations provide valuable insights into the molecular interactions underlying its biological effects.
Q9: How do structural modifications of this compound affect its biological activity?
A9: Studies investigating the relationship between procyanidin structure and activity suggest that the degree of polymerization plays a crucial role. Trimeric procyanidins like this compound generally exhibit more potent neuroprotective effects compared to monomers or dimers. [, , ] Additionally, the presence of specific functional groups, like the pyrogallol moiety found in some procyanidins, has been linked to increased cytotoxicity. []
Q10: Does the presence of galloyl moieties in this compound analogs influence their activity?
A10: Research indicates that the presence of galloyl moieties can impact the activity of procyanidins. For example, in a study comparing various procyanidins, including this compound, against Ebola virus, the presence of galloyl groups in some analogs was found to modulate their inhibitory potency. []
Q11: What are the challenges in formulating this compound for therapeutic applications?
A11: Formulating this compound presents challenges due to its sensitivity to various factors like pH, temperature, and light exposure. [, ] Additionally, its interaction with other components in formulations needs careful consideration to ensure stability and bioavailability.
Q12: Are there any strategies to improve the stability and bioavailability of this compound?
A12: Research is exploring various approaches to enhance the stability and bioavailability of this compound. Encapsulation techniques, such as using liposomes or nanoparticles, show promise in protecting the molecule from degradation and improving its delivery to target tissues. []
Q13: What in vitro models have been used to study the biological effects of this compound?
A13: Researchers have utilized various cell lines to investigate the effects of this compound. These include rat pheochromocytoma cells (PC12), human umbilical vein endothelial cells (HUVEC), human hepatoma cells (HepG2), and human intestinal cells (Caco2). [, , , , , ] These models allow for the assessment of this compound's impact on cell viability, signaling pathways, gene expression, and specific cellular functions.
Q14: Has this compound demonstrated efficacy in in vivo models of disease?
A14: Yes, this compound has shown promising results in various animal models. For instance, it exhibited neuroprotective effects in zebrafish models of Parkinson's disease, improving motor function and protecting dopaminergic neurons. [] It also alleviated obesity and improved lipid profiles in mice fed a high-fat diet. []
Disclaimer and Information on In-Vitro Research Products
Please be aware that all articles and product information presented on BenchChem are intended solely for informational purposes. The products available for purchase on BenchChem are specifically designed for in-vitro studies, which are conducted outside of living organisms. In-vitro studies, derived from the Latin term "in glass," involve experiments performed in controlled laboratory settings using cells or tissues. It is important to note that these products are not categorized as medicines or drugs, and they have not received approval from the FDA for the prevention, treatment, or cure of any medical condition, ailment, or disease. We must emphasize that any form of bodily introduction of these products into humans or animals is strictly prohibited by law. It is essential to adhere to these guidelines to ensure compliance with legal and ethical standards in research and experimentation.