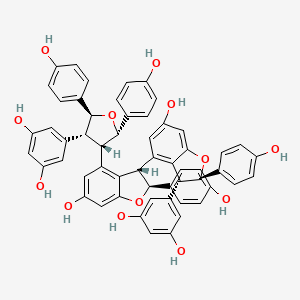
Kobophenol A
Overview
Description
Kobophenol A is a stilbenoid, specifically a tetramer of resveratrol. It can be isolated from plants such as Caragana chamlagu, Caragana sinica, and Carex folliculata seeds . The molecule has a 2,3,4,5-tetraaryltetrahydrofuran skeleton and has been shown to inhibit acetylcholinesterase .
Mechanism of Action
Target of Action
Kobophenol A, a stilbenoid and a tetramer of resveratrol, primarily targets the human angiotensin-converting enzyme 2 (ACE2) receptor and protein kinase C (PKC) . The ACE2 receptor plays a crucial role in the entry of SARS-CoV-2 into cells , while PKC is involved in various cellular functions, including cell growth and differentiation .
Mode of Action
This compound interacts with its targets by blocking the interaction between the ACE2 receptor and the spike receptor binding domain (S1-RBD) of SARS-CoV-2 . It also inhibits the activity of partially purified rat brain PKC . These interactions result in the inhibition of SARS-CoV-2 viral infection in cells .
Biochemical Pathways
The primary biochemical pathway affected by this compound is the ACE2-SARS-CoV-2 S1-RBD interaction pathway . By blocking this interaction, this compound prevents the virus from entering the host cells, thereby inhibiting the infection . The exact downstream effects of this inhibition are still under investigation.
Result of Action
The primary molecular effect of this compound’s action is the disruption of the interaction between the ACE2 receptor and the S1-RBD of SARS-CoV-2 . This disruption inhibits SARS-CoV-2 viral infection in cells . On a cellular level, this compound has been shown to exhibit anti-inflammatory activity by regulating NF-κB nuclear translocation in J774A.1 cells .
Biochemical Analysis
Biochemical Properties
Kobophenol A plays a crucial role in various biochemical reactions. It has been shown to inhibit acetylcholinesterase, an enzyme responsible for breaking down the neurotransmitter acetylcholine Additionally, this compound interacts with proteins such as Bcl-2 and Mcl-1, which are involved in regulating apoptosis . By preserving these proteins, this compound can prevent cell death induced by toxic agents like sodium nitroprusside .
Cellular Effects
This compound exhibits protective effects on various cell types. For instance, it has been shown to inhibit sodium nitroprusside-induced cell death in cardiac H9c2 cells by suppressing the activation of c-Jun NH2-terminal kinase and p38 mitogen-activated protein kinase . This compound also influences cell signaling pathways, such as the NF-κB pathway, by inhibiting the translocation of NF-κB into the nucleus in macrophage cells . These actions help reduce inflammation and protect cells from oxidative stress.
Molecular Mechanism
At the molecular level, this compound exerts its effects through several mechanisms. It binds to and inhibits acetylcholinesterase, preventing the breakdown of acetylcholine . Additionally, it inhibits the activation of c-Jun NH2-terminal kinase and p38 mitogen-activated protein kinase, which are involved in stress response pathways . This compound also modulates gene expression by inhibiting the NF-κB pathway, reducing the production of pro-inflammatory cytokines .
Temporal Effects in Laboratory Settings
In laboratory settings, the effects of this compound have been observed to change over time. For example, its protective effects against sodium nitroprusside-induced cell death in cardiac H9c2 cells are sustained over a period of treatment . The stability and degradation of this compound in vitro have not been extensively studied, but its long-term effects on cellular function suggest that it remains active over extended periods.
Dosage Effects in Animal Models
The effects of this compound vary with different dosages in animal models. At lower doses, it has been shown to provide protective effects against cell death and inflammation
Metabolic Pathways
This compound is involved in several metabolic pathways. It interacts with enzymes such as acetylcholinesterase and proteins like Bcl-2 and Mcl-1, influencing metabolic flux and metabolite levels
Transport and Distribution
Within cells and tissues, this compound is likely transported and distributed through interactions with specific transporters and binding proteins. While detailed studies on its transport mechanisms are limited, its interactions with cellular proteins suggest that it may be actively transported to specific cellular compartments where it exerts its effects .
Subcellular Localization
This compound’s subcellular localization is crucial for its activity. It has been shown to inhibit the nuclear translocation of NF-κB in macrophage cells, indicating that it may localize to the cytoplasm and nucleus
Preparation Methods
Kobophenol A can be synthesized through various methods. One notable method involves the acid-catalyzed epimerization of this compound to carasinol B . This process provides important information about the biotransformation of this compound in plants and its metabolism in rats . Industrial production methods typically involve extraction from natural sources, followed by purification processes to isolate the compound .
Chemical Reactions Analysis
Kobophenol A undergoes several types of chemical reactions:
Oxidation: It can be oxidized to form various derivatives.
Reduction: Reduction reactions can modify its structure, potentially altering its biological activity.
Substitution: this compound can undergo substitution reactions, where functional groups are replaced by others.
Common reagents and conditions used in these reactions include acid catalysts for epimerization and various oxidizing and reducing agents . Major products formed from these reactions include carasinol B and other resveratrol derivatives .
Scientific Research Applications
Kobophenol A has a wide range of scientific research applications:
Chemistry: It is studied for its unique chemical structure and reactivity.
Industry: This compound is used in the development of bioactive compounds and natural product libraries.
Comparison with Similar Compounds
Kobophenol A is unique due to its tetrameric structure and its ability to inhibit multiple biological targets. Similar compounds include:
Resveratrol: A monomeric stilbenoid with antioxidant properties.
Piceatannol: A resveratrol derivative with anti-inflammatory and anticancer properties.
Viniferin: A resveratrol trimer with antimicrobial and anticancer activities.
This compound stands out due to its higher molecular complexity and broader range of biological activities .
Properties
IUPAC Name |
5-[(2S,3R,4S,5S)-4-[(2S,3S)-3-[(2R,3R)-3-(3,5-dihydroxyphenyl)-6-hydroxy-2-(4-hydroxyphenyl)-2,3-dihydro-1-benzofuran-4-yl]-6-hydroxy-2-(4-hydroxyphenyl)-2,3-dihydro-1-benzofuran-4-yl]-2,5-bis(4-hydroxyphenyl)oxolan-3-yl]benzene-1,3-diol | |
---|---|---|
Source | PubChem | |
URL | https://pubchem.ncbi.nlm.nih.gov | |
Description | Data deposited in or computed by PubChem | |
InChI |
InChI=1S/C56H44O13/c57-33-9-1-27(2-10-33)53-47(31-17-37(61)21-38(62)18-31)49-43(23-41(65)25-45(49)67-53)52-50-44(24-42(66)26-46(50)68-55(52)29-5-13-35(59)14-6-29)51-48(32-19-39(63)22-40(64)20-32)54(28-3-11-34(58)12-4-28)69-56(51)30-7-15-36(60)16-8-30/h1-26,47-48,51-66H/t47-,48+,51-,52+,53+,54-,55-,56-/m1/s1 | |
Source | PubChem | |
URL | https://pubchem.ncbi.nlm.nih.gov | |
Description | Data deposited in or computed by PubChem | |
InChI Key |
RAUCCLKIJHMTND-LUPMIFTGSA-N | |
Source | PubChem | |
URL | https://pubchem.ncbi.nlm.nih.gov | |
Description | Data deposited in or computed by PubChem | |
Canonical SMILES |
C1=CC(=CC=C1C2C(C(C(O2)C3=CC=C(C=C3)O)C4=C5C(C(OC5=CC(=C4)O)C6=CC=C(C=C6)O)C7=C8C(C(OC8=CC(=C7)O)C9=CC=C(C=C9)O)C1=CC(=CC(=C1)O)O)C1=CC(=CC(=C1)O)O)O | |
Source | PubChem | |
URL | https://pubchem.ncbi.nlm.nih.gov | |
Description | Data deposited in or computed by PubChem | |
Isomeric SMILES |
C1=CC(=CC=C1[C@@H]2[C@H]([C@H]([C@H](O2)C3=CC=C(C=C3)O)C4=C5[C@@H]([C@H](OC5=CC(=C4)O)C6=CC=C(C=C6)O)C7=C8[C@H]([C@@H](OC8=CC(=C7)O)C9=CC=C(C=C9)O)C1=CC(=CC(=C1)O)O)C1=CC(=CC(=C1)O)O)O | |
Source | PubChem | |
URL | https://pubchem.ncbi.nlm.nih.gov | |
Description | Data deposited in or computed by PubChem | |
Molecular Formula |
C56H44O13 | |
Source | PubChem | |
URL | https://pubchem.ncbi.nlm.nih.gov | |
Description | Data deposited in or computed by PubChem | |
DSSTOX Substance ID |
DTXSID00333128 | |
Record name | Kobophenol A | |
Source | EPA DSSTox | |
URL | https://comptox.epa.gov/dashboard/DTXSID00333128 | |
Description | DSSTox provides a high quality public chemistry resource for supporting improved predictive toxicology. | |
Molecular Weight |
924.9 g/mol | |
Source | PubChem | |
URL | https://pubchem.ncbi.nlm.nih.gov | |
Description | Data deposited in or computed by PubChem | |
CAS No. |
124027-58-3 | |
Record name | Kobophenol A | |
Source | CAS Common Chemistry | |
URL | https://commonchemistry.cas.org/detail?cas_rn=124027-58-3 | |
Description | CAS Common Chemistry is an open community resource for accessing chemical information. Nearly 500,000 chemical substances from CAS REGISTRY cover areas of community interest, including common and frequently regulated chemicals, and those relevant to high school and undergraduate chemistry classes. This chemical information, curated by our expert scientists, is provided in alignment with our mission as a division of the American Chemical Society. | |
Explanation | The data from CAS Common Chemistry is provided under a CC-BY-NC 4.0 license, unless otherwise stated. | |
Record name | Kobophenol A | |
Source | EPA DSSTox | |
URL | https://comptox.epa.gov/dashboard/DTXSID00333128 | |
Description | DSSTox provides a high quality public chemistry resource for supporting improved predictive toxicology. | |
Disclaimer and Information on In-Vitro Research Products
Please be aware that all articles and product information presented on BenchChem are intended solely for informational purposes. The products available for purchase on BenchChem are specifically designed for in-vitro studies, which are conducted outside of living organisms. In-vitro studies, derived from the Latin term "in glass," involve experiments performed in controlled laboratory settings using cells or tissues. It is important to note that these products are not categorized as medicines or drugs, and they have not received approval from the FDA for the prevention, treatment, or cure of any medical condition, ailment, or disease. We must emphasize that any form of bodily introduction of these products into humans or animals is strictly prohibited by law. It is essential to adhere to these guidelines to ensure compliance with legal and ethical standards in research and experimentation.